L. P. Dyrud,
M. M. Oppenheim,
G. Vetoulis,
A. F. vom Endt
Center for Space Physics
Boston University
Abstract:
Micro-meteor trails are frequently observed in the ionosphere, and their radar echos have long been used to infer neutral wind velocities and temperature, meteor trail size, and altitude. We present the first computer simulations and analyses of the plasma dynamics of meteor trails. A gradient drift instability on the edges of these trails develops into turbulence in ~1-5 ms. This turbulence causes anomalous perpendicular diffusion that increases with trail altitude and density gradient. We find that meteor trail motion in the equatorial E-region is controlled by turbulent plasma processes, and their radar signals may not easily be used to infer neutral atmosphere properties.
Introduction
This study investigates the small scale electro-dynamics of weakly ionized meteor trails in the ionosphere. The Earth is continually bombarded by dust to sand grain size meteors [Ceplechaet al., 1998]. The meteors evaporate their atoms during atmospheric entry and these hot atoms generate a cylinder of ionization roughly 100 times more dense than the surrounding ionospheric plasma. To better understand the evolution of plasma columns we present hybrid plasma simulations that have revealed a number of previously unknown dynamics. Simulations of meteor trails in the equatorial ionosphere showed that a gradient drift Farley-Buneman [Fejer et al., 1975, Chapin et al., 1998] (GDFB) instability develops quite readily where the trail density gradient and the electric field are the largest. The development of this instability has a number of important implications for the interpretation of meteor trail radar echos. We find, that the instability increases the perpendicular rate of diffusion by, in some cases, nearly an order of magnitude. Altered diffusion rates for meteor trails have the following implications. Meteor trail diffusion is used to infer atmospheric temperatures. The initial radius and altitude calculations of meteor trails are often based upon their diffusion rates [Baggaley et al., 1980]. These findings of increased perpendicular diffusion modify previous work [Jones et al., 1991] who theoretically demonstrated that the diffusion of meteor trails perpendicular the geomagnetic field is greatly inhibited.
The Numerical Simulator
- Use a hybrid plasma simulator with (PIC) ions and fluid electrons.
- Simulate the meteor trail as Gaussian distribution of increased density.
- Conduct 2-D simulations in the plane perpendicular to the geomagnetic field.
- Employ periodic boundary conditions, thus in some cases treating the trail as infinitely long.
The Simulations
The simulations are conducted in the plane perpendicular to the background magnetic field,where the instability primarily occurs. Although interesting dynamics in the direction parallel to the magnetic field exist, we argue that the trail will primarily develop according to standard ambipolar diffusion theory. The meteor trail is initialized as a Gaussian distribution of increased density. The ion, and electron velocities, and electric field are initialized according to a steady state solution of the fluid equations [Oppenheim et al., 2000]. The simulations were conducted using parameters consistent with the ionospheric conditions found at altitudes from 105 km to 110 km with data taken from the IRI90 [Blitza, 1990] and CIRA72 [Rees, 1986] models for the ionosphere and neutral atmosphere with collision frequencies from Banks and Kockarts. [1973]. Figure 1 shows simulations of meteor trails with axis perfectly aligned with the geomagnetic field.
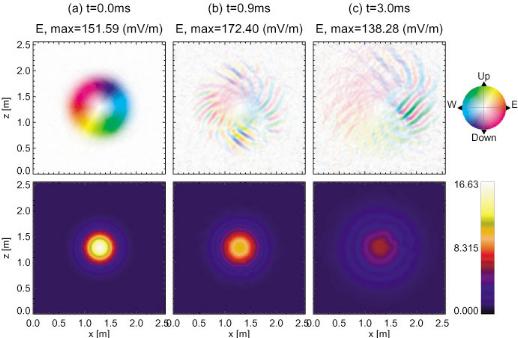
Trail Evolution at 105 km Altitude
Figure 1
Vector electric field (E) in mV/m, and the density ratio of the trail plasma to the background density ntrail/ nbackground in a log scale at three different times during the simulation. The direction and magnitude of E is given by the color wheel with magnitude increasing at larger radii. The axis are the plane that is both perpendicular to the trail axis and magnetic field. Panels (a) are the initial stages of the simulation, panels (b) are at 1 ms and show that the GDFB has already developed strong ripples in the trail density. Panels (c) are at only three ms, yet the instability is fully developed and the peak density is already been reduced to ~1/3 of the original value.
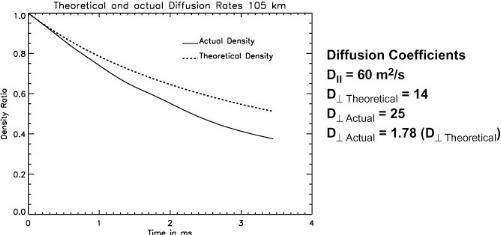
Trail Evolution at 105 km Altitude
Figure 2
Density ratio vs. time (ms) for the simulation using 105 km altitude parameters. The density ratio is the value of density at the full-width half-max of the initial Gaussian distribution divided by the initialized density.
Summary
- Increased diffusion has implications for the use of diffusion rates as radar echo altitude measures, and may help explain the factor of two discrepancy between observed and theoretical calculations of initial trail radii.
- Anomalous diffusion also raises problems for the use of meteor trails for neutral temperature measurements.
- Hybrid plasma simulations show that a Gradient Drift Farley-Buneman (GDFB) rapidly develops on meteor trails in the ionosphere radically altering the trails evolution.
- Anomalous perpendicular diffusion of meteor trails that increases with increasing altitude.