Focused Research Program Faculty Member Uses Computational Models to Design Better Batteries
BY GINA MANTICA
Every time you plug in your cell phone, tiny lithium ions travel back to the battery’s surface and give it the charge, or energy, that it needs to power on. But if the battery’s surface isn’t perfectly flat, spiky growths of metal called dendrites can form and decrease the battery’s efficiency or worse, can cause short circuiting. Researchers at Boston University use machine learning to study the chemistry and physics underlying the growth of dendrites and design better batteries.
Emily Ryan, a Research Fellow at the Hariri Institute and Associate Professor in Mechanical Engineering, developed a computational model to build more efficient, rechargeable lithium metal batteries. Her lab’s findings were published recently in The Journal of Chemical Physics.
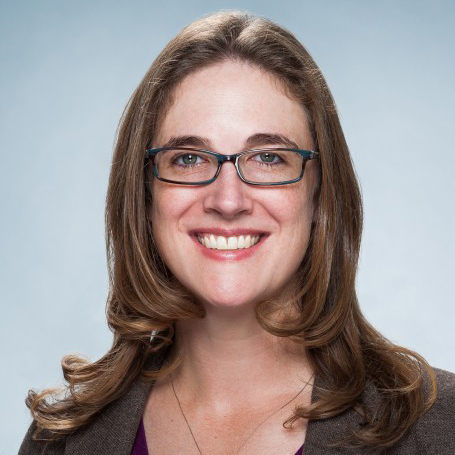
The project was supported by the Hariri Institute’s Machine Learning for Chemistry & Materials Science Focused Research Program, which aims to advance the design and synthesis of small molecules and materials through machine learning. “With modeling, we can ask questions otherwise challenging on real surfaces. For batteries, we can play around with physics to see what phenomena are causing dendrite growth,” said Ryan.
Batteries have three main parts: an anode, or negative end, a cathode, or positive end, and a material in the middle called the electrolyte that allows ions to flow between the anode and cathode. The anode surface is where ions are released into the electrolyte and in rechargeable batteries, these particles are restored to the anode during charging.
Rechargeable batteries are better for the environment than other forms of energy, but currently they cannot store enough energy to meet rising demands. Improving efficiency requires changing the design of a battery’s anode to prevent dendrite growth, which is hard to do since the chemical and physical reactions happening there are too small to be seen by even the best microscopes. Computational modeling enables researchers to study and manipulate the reactions happening on the anode to optimize a battery’s design.
Playing around with the physical structure of the anode’s surface is one way that researchers can improve a battery’s efficiency. Ryan’s team used their model to test how different sized holes on the anode’s surface affect the growth of dendrites. The researchers found that narrow cavities lead to less dendrite growth, while wide depressions lead to more growth. This suggests that the geometry of the anode’s surface can affect the growth of dendrites, and in turn the battery’s efficiency. “Could I design a surface that has a pattern that allows me to get exactly what I want,” reflected Ryan. Designing a better battery could entail controlling imperfections on the anode, like the width of indentations, to improve efficiency.
Ryan’s team also examined how the chemical makeup of the anode’s surface impacts dendrite growth using their model. If lithium metal is exposed due to cracks or differences in battery design, the surface can be highly reactive. The researchers found that reactivity prevented dendrite growth in wider holes on the anode’s surface. However, reactivity spurred dendrite growth in narrow cavities. This suggests an interplay between the chemical and physical composition of the anode in determining the growth of dendrites and ultimately, the battery’s efficiency. “What we want people to understand is that it’s critical to understand both the reactivity and surface properties since they are interrelated,” said Ryan.
Ryan’s computational approach to studying surface-level interactions can be used to improve other types of batteries, fuel cells, and even manufacturing processes. Efficiency in these systems doesn’t have to mean perfection, though. As exemplified in the researchers’ study, some “imperfections” can actually make systems more efficient. “We can never get a perfect surface,” said Ryan, “But can we engineer a surface with specific defects to control growth?”
To learn more about the Hariri Institute’s transformational research, click here to sign up for our newsletter.