Tagged: agriculture
Not Your Old McDonald’s Farm
Vertical farms could provide the food of the future.
According to a growing body of evidence, the human species may be in crisis. Earth is becoming overpopulated, polluted, and drained of resources at an alarming rate. The basic issue is simply about numbers: there are a whole lot of us, and not much land. The planet is currently populated by 7 billion human beings, and projected to rise to 9.5 billion people by 2050.1 In order to feed the growing population, we would need an area of additional farmland approximately the size of Brazil.2 Yet we cannot create much more arable land than we have. The productivity of plant life may already be at maximum capacity even as we try to increase crop yield year after year.3 We need to work with what we have already taken to meet our needs.
Unfortunately, we are not using our resources wisely. Commercial agricultural practices typically have high-energy costs due to irrigation methods, fertilizer, and fuel usage. As the cost of fuel increases, so does the market cost of food. While products like corn ethanol reduce fuel costs in the short term, this means more of our limited crop supply is not available for consumption and may further increase the cost of food. The current system also forces us all to depend on a select few countries to produce enough to feed the whole world, causing high shipping costs, and one poor growing season can place dire limitations on all of us.4
Furthermore, current irrigation methods waste more water than any other human activity. Between 70% and 90% of the world’s freshwater supply (which is a mere 3% of all water to begin with) is used for irrigation of farmland and then rendered unsanitary for human use due to pesticides.2 On top of these issues, modern agricultural practices cause high outputs of pollutants in our air and water and lead to food-borne illnesses due to unsanitary animal overcrowding. Our only hope is to change the way we feed ourselves, to reduce waste and to maximize efficiency without increasing our consumption of materials. What we need is another agricultural revolution.
Solving a Growing Problem
The vertical farm is a potential solution for these global issues. Ideally, a vertical farm would be a large, independently operating structure centrally located in a major city. It would feature two multistory, skyscraper-like buildings working together- one to manage food production with nutrient film techniques, and another to manage waste through living machines and generate energy with photovoltaic cells and carbon sequestering. Popularized in recent years by Dr. Dickson Despommier, a professor at Columbia University, the concept originated in the 1950’s with a “Glass House” and has been further developed by several innovators over the years.5 Controlled Environment Agriculture (CEA), which allows for control of temperature, pH, and nutrients, has also been employed in for many years in commercial greenhouses in order to produce crops unsuited for the local climate. Although these greenhouses are often high-yielding, they typically require fossil fuels that produce considerable emissions, and do not eliminate agricultural runoff. In contrast, the vision of the vertical farm is one of grand scale: sky-scraping, glass-paneled buildings placed in every major urban center to provide affordable, carbon neutral, pollutant-free food to the cities’ residents. A project of this scale involves a huge number of factors, all dependent on the ratio of cost to potential yield. This could completely change the way we get food from the ground to the table. Instead of shipping produce from several states away, or from outside of the country, grocery stores could stock fruits and vegetables grown right in the heart of their city. It would reduce pollution, increase production, and be healthier for ourselves and the planet.
Designing the Future Farm
Constructing vertical farms within major cities may eliminate the problems of land shortage, pollution, deforestation, water shortage, and unsanitary practices commonly found in the agriculture industry around the world. Office spaces could be located nearby for the business and management end of the operation. This design would have high initial costs, but over time would recoup these losses and become highly profitable while benefiting urban centers and the environment. Savings from reduced energy and maintenance costs would over time compensate for initial losses, and sales profits are projected to be comparable to stock market averages.2 Despite high costs for technology and construction at the upstart, vertical farms could have astounding effects on local and global populations. The cleanliness and convenience of an environmental friendly food center would improve the property value of surrounding urban neighborhoods and improve quality of life. It would be an economic boon to cities and generate a wide range of new urban jobs, but would cause employment and sales losses for rural farmers. Global effects could be even more important. This design would be especially effective in tropical and subtropical locations, where incoming solar radiation is at a maximum and controlled climates are easiest to maintain. If implemented in less developed nations in these locations, vertical farms could transform those economies and be a catalyst to slow excessive population growth as urban agriculture is adopted as a strategy for sustainable food production. It might also reduce or eliminate the occurrence of armed conflict over natural resources, such as water and land, as both would be more available thanks to successful conservation.
Putting the Design to Work
Vertical farming proposes to be the ultimate design for sustainability and conservation of resources. A controlled climate allows for high yield, year-round crop production. Consider strawberries as an example: 1 acre of berries grown indoors produces as much fruit in one year as 30 outdoor acres.2 Generally, growing indoor crops is four to six times more productive than outdoor farming. This method also protects plants against inclement weather, parasites, and disease, so fewer crops are lost and toxic chemicals are not needed for pesticides. Using special dirt-free hydroponic systems and re-circulating ‘living machines’, we can even recycle city waste water and turn it into clean water for irrigation.6 This method of water use would drastically reduce consumption, eliminate most pollutants found in runoff, and lead to cleaner rivers, lakes and oceans. Additionally, a vertical farm could add energy “back to the grid”, rather than consuming nonrenewable fossil fuels. Tractors, plows and shipping trucks, all “gas guzzlers”, would be unneeded in a vertical farm. A combination of solar panels and sequestered methane generated from the composting of non-edible organic materials could generate the heat necessary for a controlled climate.7 Essentially, the building could run on sunshine and garbage. Waste from other parts of the city could even be reduced if we were able to incorporate it for methane generation in our farm. Vertical farming might be our answer for low waste, high yield farming of the future.
However, we likely won’t see this solution implemented any time soon. While technologically possible today, urban vertical farms are unlikely to find a place in society in the near future because of the finances required to begin this endeavor. Hope lies with universities and private institutions to expand the idea with further research and with far-sighted investors to provide the funds to implement this incredible solution. Perhaps with more information, a corporation could be convinced to finance the first commercial vertical farm.
References
1 United Nations, Department of Economic and Social Affairs, Population Division (2011): World Population Prospects: The 2010 Revision. New York.
2 Despommier, Dickson D. The Vertical Farm: Feeding Ourselves and the World in the 21st Century. New York: Thomas Dunne, 2010. Print.
3 Li, Sophia. “Has Plant Life Reached Its Limits?” New York Times. New York Times, 20 Sept. 2012. Web. 15 Oct. 2012. .
4 Lowrey, Annie. “Experts Issue a Warning as Food Prices Shoot Up.” The New York Times. The New York Times, 4 Sept. 2012. Web. 15 Oct. 2012. .
5 Hix, John. 1974. The glass house. Cambridge, Mass: MIT Press.
6 Ives-Halperin, John and Kangas, Patrick C. 2000. 7th International Conference on Wetland Systems for Water Pollution Control. International Water Association, Orlando, FL. pp. 547-555. http://www.enst.umd.edu/files/secondnature.pdf
7 Concordia University (2011, October 4). From compost to sustainable fuels: Heat-loving fungi sequenced. ScienceDaily. Retrieved October 18, 2012, from http://www.sciencedaily.com/releases/2011/10/111003132441.htm.
The Plant Horror Story
Death and destruction in the developing world - are plants to blame?
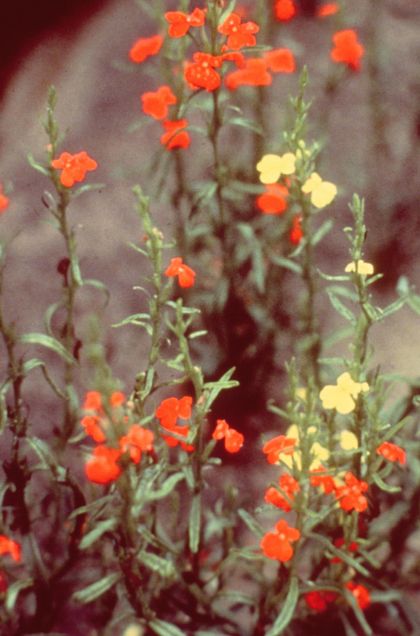
Striga asiatica is a parasitic plant native to Africa. Image credit | Bugwood.org via Wikimedia Commons
The media often mentions atrocities committed in developing countries. Most of these crimes are attributed to vicious dictators, nasty civil wars, poor infrastructure, and ignorance of the people. However, one of the main culprits, one guilty of causing countless cases of malnutrition, starvation, and poverty, is not human, but plant. Plants are generally thought of as passive organisms that create an ambient and stable environment. However, many people do not know that plants can be parasitic. These parasitic plants generally target other plants from which they absorb nutrients necessary for growth and survival. One of the most widespread and deadly parasites is the Striga genus of plant parasites of whichStriga asiatica causes some of the greatest damage. Striga asiatica is native to Sub-Saharan Africa and Asia, places where malnutrition, starvation, and poverty are rampant and most farmers are subsistence farmers.
The Killer
Striga asiatica is a beautiful flowering plant, but its real nature is truly deadly for many farmers. In fact its harm to farmers can be seen through the name given to it by American farmers after it was briefly introduced to the United States - witchweed. Its seeds are very small and almost indiscernible by the naked human eye. Not only is it almost impossible to see, but it can live dormant in the earth for up to 20 years while waiting for a signal from the host in order to initiate germination. Striga asiatica growth is initiated by chemical signals from hosts, which are usually types of grasses such as corn and sorghum. Once the plant has parasitized it host, host growth is severely stunted. Striga asiatica is known to reduce crop yields by up to 90%, and also releases thousands of hardy seeds to cause the cycle again.1
Current Prevention Techniques
One of the major policies that has been undertaken to stop the agricultural yield losses due to this parasite is the attempt to establish better farming practices. One way to do this is “intercropping,” wherein Desmodium, a type of legume, is grown along with Striga asiatica. The properties of Desmodium allow it to suppress Striga asiatica growth while allowing crops to grow.2 Another approach has been to breed a Striga asiatica resistant crop. This research has focused mainly on Zea mays (corn) and some very promising finds have been made. In fact a strain of Zea mays known as TMV-1 resulted in only a 13.3% yield loss.3 Obtaining the ability to use specialized corn, however, is often expensive and an unlikely alternative for subsistence farmers in many of the afflicted regions.
Other approaches have focused on herbicides. A promising and inexpensive type of herbicide known as acetolactate synthase (ALS)-inhibiting herbicides has been shown to suppress Striga asiatica growth by 75-95%.4 However, these approaches have been difficult to implement and do not completely eradicate the problem posed by the parasite. Therefore, the most promising research that is being conducted in regards to Striga asiatica focuses on the plant-plant small molecule signaling that occurs between Striga asiatica and its host, which can theoretically provide a permanent solution.
Investigation and Future Plants
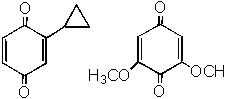
CPBQ (left) and DMBQ (right) are two compounds that help stimulate premature growth of Striga asiatica.
This plant-plant small molecule signaling model is known as semagenesis and has a wide variety of potential impacts. Research into communication between Striga asiatica and its host has led to the identification of numerous germination stimulants. These stimulants are mainly quinones, which are common organic cyclic structures that act as oxidizing agents, such as 2,6-dimethoxy-1,4-benzoquinone (DMBQ) and cyclopropyl-p-benzoquinone (CPBQ). By identifying these stimulants, scientists have been able to develop synthetic germination stimulants similar to these quinones. Since DMBQ analogs have been able to induce germination without the presence or organic products of a host, this cheap germination stimulant could be used to preemptively induce growth of the parasite.5 Without the presence of the host plants, the parasites can only live about a week. After this period, crops can be planted without suffering a reduced yield.
Further research has found other molecules that have a large effect on Striga asiatica growth, and even plant growth in general.6 These molecules include monolignols, which are the precursors to lignin, the compound that makes up the secondary cell wall of plants. Monolignols are also the oxidative precursors to germination stimulating benzoquinones and other highly reactive oxygen species. These highly reactive compounds are known for causing symptoms of aging and are the reason why food companies inundate consumers with antioxidant labels. This study provides an intriguing preview into the evolution of plants because it shows that all plants, not just parasitic plants, have pathways relevant to parasitism.
Even more recent experiments have focused on using RNAi, or RNA interference, which allows regulation of gene activity. Specifically, genes can be downregulated or knocked out in order to identify the plant parasitism mechanisms and the genes responsible for the parasite’s growth. The greatest potential use for this research is for the creation of anti-parasitism measures.
Plants have already been used to create many drugs and medical therapies. Continued research of parasitic plants using RNAi could give new insights into human metabolic pathways, genetic mutations, and countless other mechanisms. Most importantly, we may be able to develop methods of manipulating plant parasitism in developing countries, which would benefit the most from a boost in agricultural production.
The next time you hear about the food crises occurring in Africa and Asia, remember that the people who solve many of the issues plaguing these regions are not only politicians and social workers, but even scientists.
References
1Cochrane, V., & Press, M. C. (1997, May). Geographical Distribution and Aspects of the Ecology of the Hemiparasitic Angiosperm Striga asiatica (L.) Kuntze: A Herbarium Study. Journal of Tropical Ecology, 13, 371-380.
2Khan, Z. R., Pickett, J. A., Wadhams, L. J., Hassanali, A., & Midega, C. A. (2006, September). Combined control of Striga hermonthica and stemborers by maize-Desmodium spp. intercrops. Crop Protection, 25, 989-995.
3Mbwaga, A. M., & Massawe, C. (2001, February). Evaluation of maize cultivars for striga resistance in the Eastern Zone of Tanzania. Seventh Eastern and Southern Africa Regional Maize Conference, 174-178.
4Abayo, G. A., English, T., Eplee, R. E., Kanampiu, F. K., Ransom, J. K., & Gressel, J. (1998, July). Control of parasitic witchweeds (Striga spp.) on corn (Zea mays) resistant to acetolactate synthase inhibitors. Weed Science, 46, 459-466.
5Palmer, A. G., Gao, R., Maresh, J., Erbil, W. K., & Lynn, D. G. (2004). Chemical biology of multi-host/pathogen interactions: chemical perception and metabolic complementation. Annual Review of Phytopathology, 42, 439-464.
6Palmer, A. G., Chen, M. C., Kinger, N. K., & Lynn, D. G. (2009, May). Parasitic angiosperms, semagenesis and general strategies for plant-plant signaling in the rhizosphere. Pest Management Science, 65, 512-519.
7Nickrent, D.L. (Photographer). Scrophulariaceae [Photograph], Retrieved April 3, 2009, from: http://www.life.uiuc.edu/plantbio/digitalflowers/Scrophulariaceae/27.htm.
The Effect of Wind Energy Development on Bats
Is wind energy really as “green” as we think? More