The Line Between Biology and Technology Has Blurred—There’s No Going Back
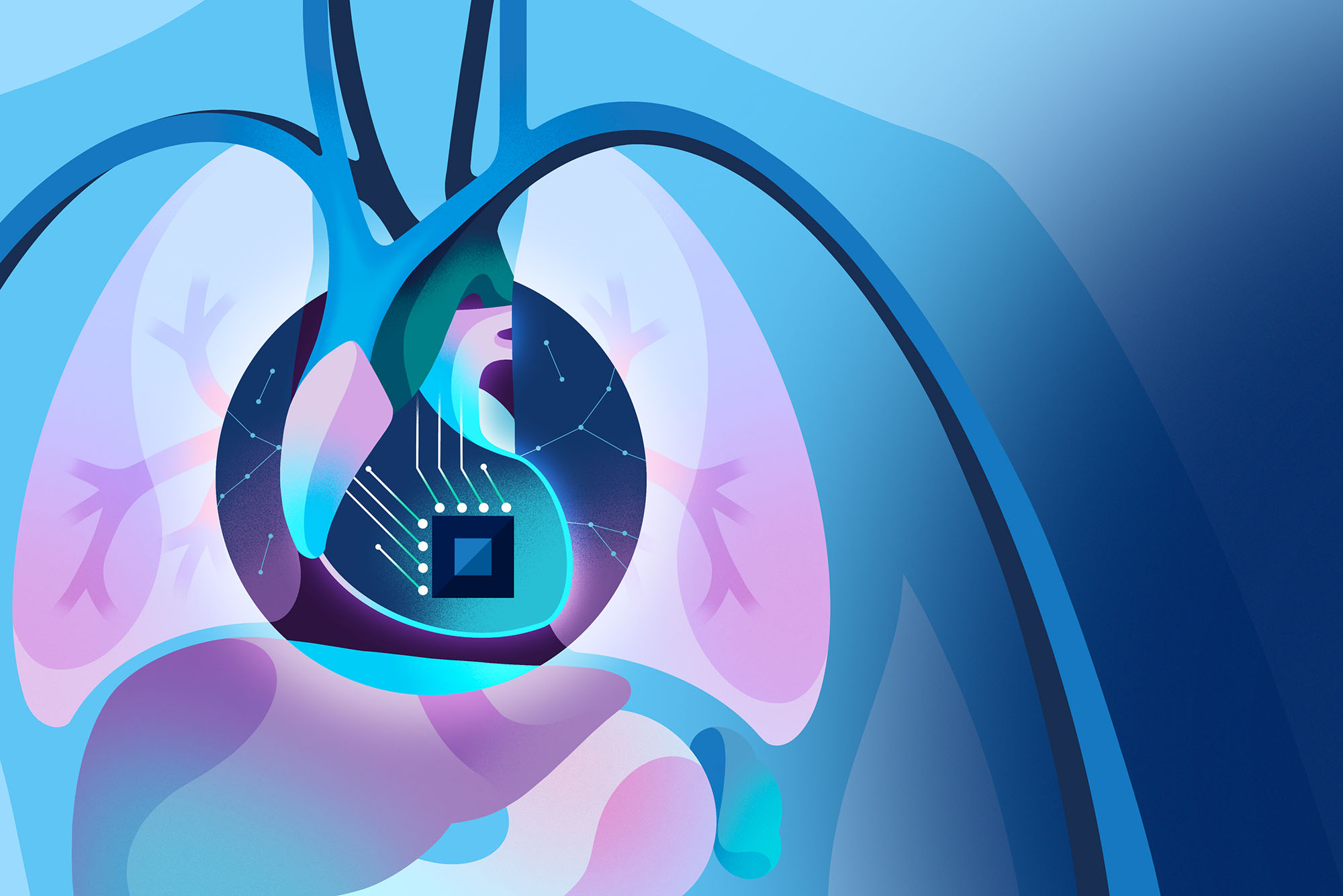
The Line Between Biology and Technology Has Blurred—There’s No Going Back
BU scientists reflect on how this interface has changed the world and on the potential of artificial intelligence, synthetic biology, and biomedical engineering
How many times today has technology helped make decisions for you?
Have you used Waze to route you from point A to point B as quickly as possible? Have you asked Alexa or Siri to suggest some music, or has autocorrect improved spelling in your texts or emails? Do you ever stop to think about the influence these technologies have over your decisions and behaviors, or have these technologies simply blended seamlessly into the way you interact with the world?
“Just think about how many times a day you collaborate with an artificially intelligent agent,” says Kate Saenko, a College of Arts & Sciences associate professor of computer science and director of BU’s master’s program in artificial intelligence (AI). “We are using technology to assist us with little jobs and tasks all the time.”
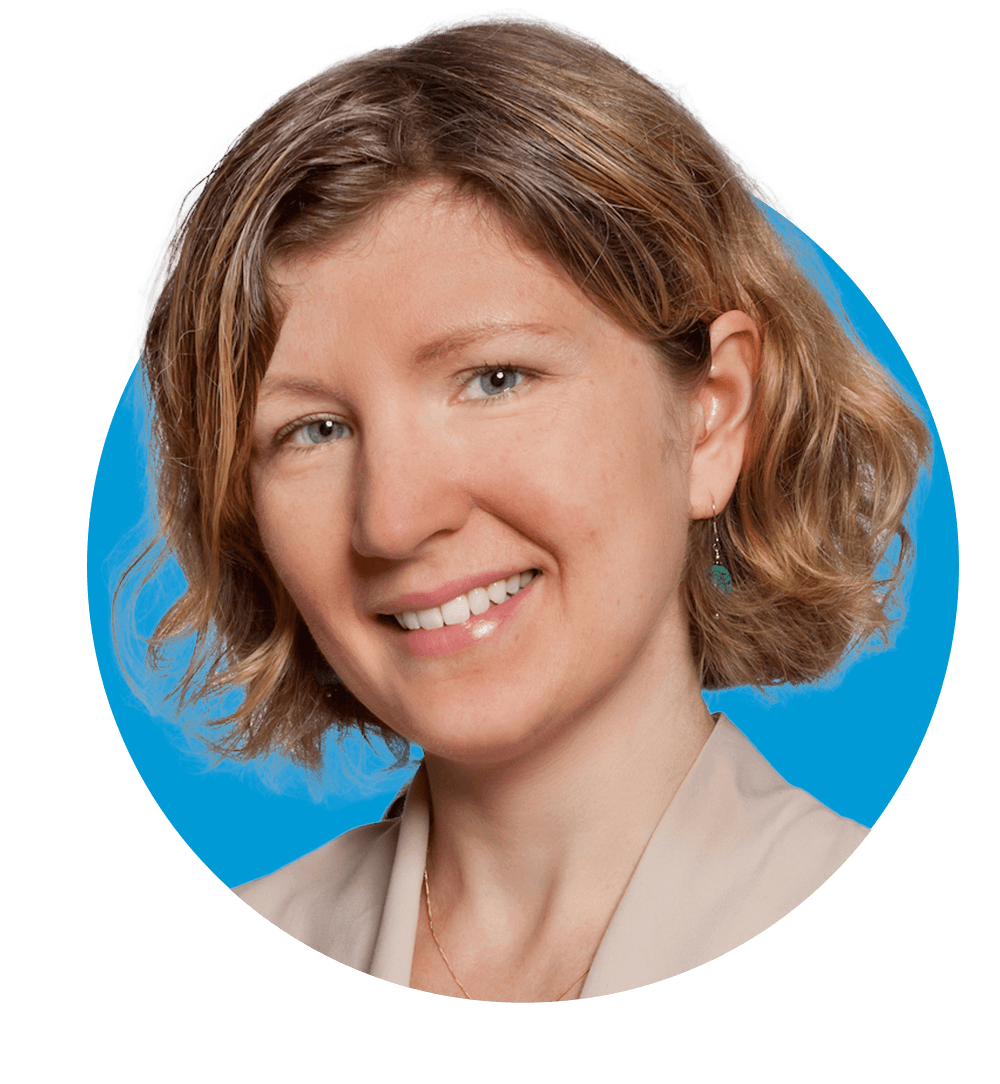
Although not all of these technologies are truly artificially intelligent, Saenko says we can expect them to get closer and closer to mimicking human decision-making as time goes on. When we get to that point, she wonders, will we humanize those AIs or will we still think of them as technologies?
“We draw a lot of inspiration from human learning and intelligence—that’s the only model we know, and that’s what we’re trying to replicate with AI,” she says. “The most common approach we use now, an artificial neural network, can be designed to interpret complex data, such as deciphering the meaning of a paragraph.”
These AI networks are getting bigger and more powerful, but they still pale in comparison to their source of inspiration. Saenko says the company NVIDIA has developed a neural model—the biggest such network yet created—that it claims has one trillion connections. In contrast, the number of neural connections in the human brain is estimated at 100 trillion.
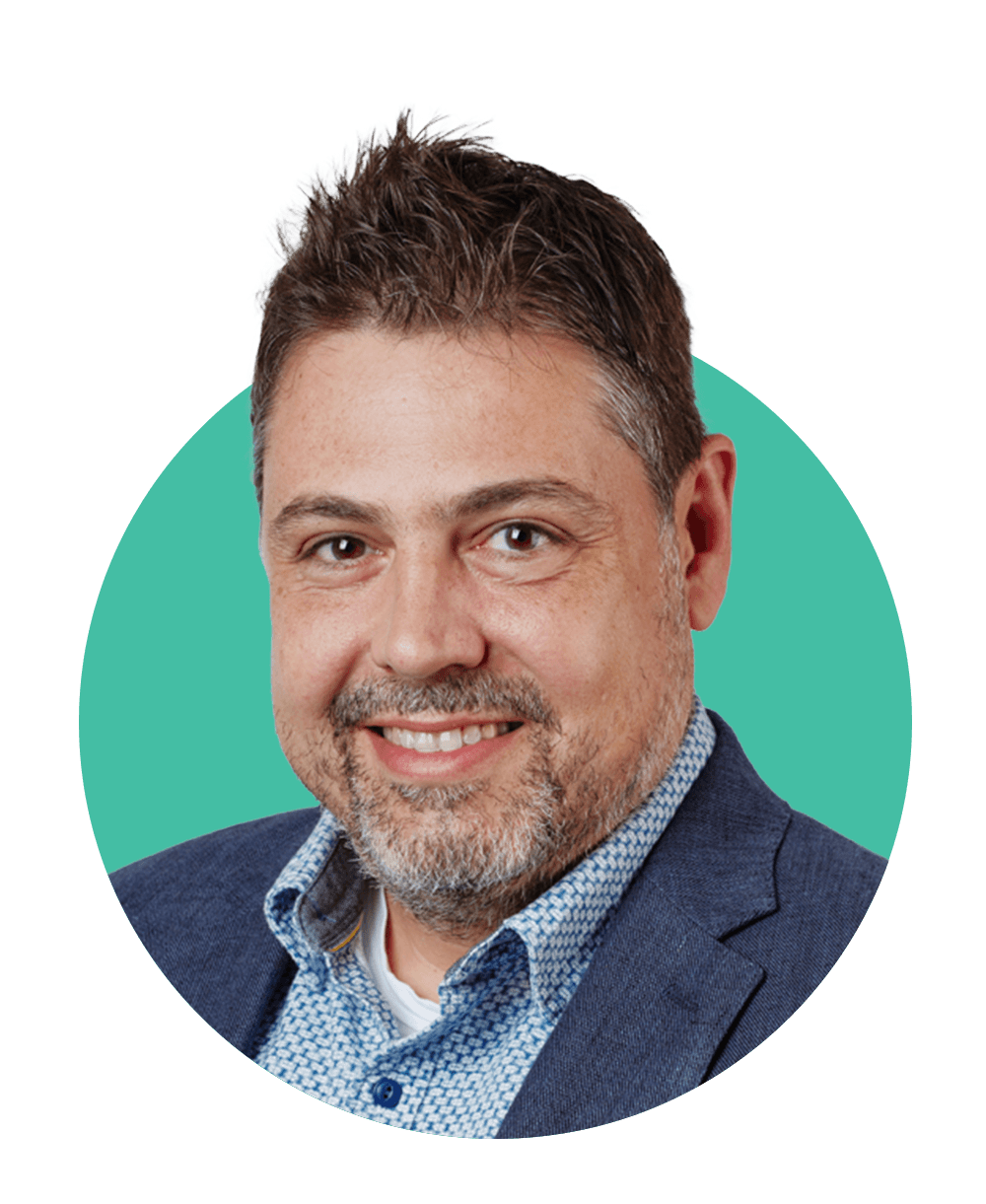
For AI to continue to grow and become more sophisticated, scientists will first need to learn more about the workings of human brains. “We’re still trying to figure out how this thing works between our own ears,” says Basilis Zikopoulos, a College of Health & Rehabilitation Sciences: Sargent College and School of Medicine associate professor of health sciences and director of Sargent’s Human Systems Neuroscience Laboratory. He and other BU researchers are mapping out the neural connections of human brains and using those data to build an artificially intelligent computer model.
“This will be another tool we use to understand how the human brain changes in development,” Zikopoulos says. “Once we can look at all the data in a computer simulation of a human brain, then we will be able to start disrupting the model and see how it responds in comparison to people who we know have sleep disorders, autism, schizophrenia, depression, etc.”
Zikopoulos hopes that ultimately the simulated brain could be used by clinicians to model patients’ brains in order to diagnose psychological disorders and brain diseases and to design and test the effects of personalized treatments or interventions to improve brain health and well-being in patients.
“Once you have a better idea of the structural mechanisms in the brain causing disorder, you can figure out which therapies or interventions might have an effect,” he says. He imagines such interventions could include deep brain electrode stimulation or drugs that impact brain activity. However, before a simulated brain could ever be sophisticated enough to provide that level of insight and prediction into an individual patient’s brain, new ethical ground would have to be covered.
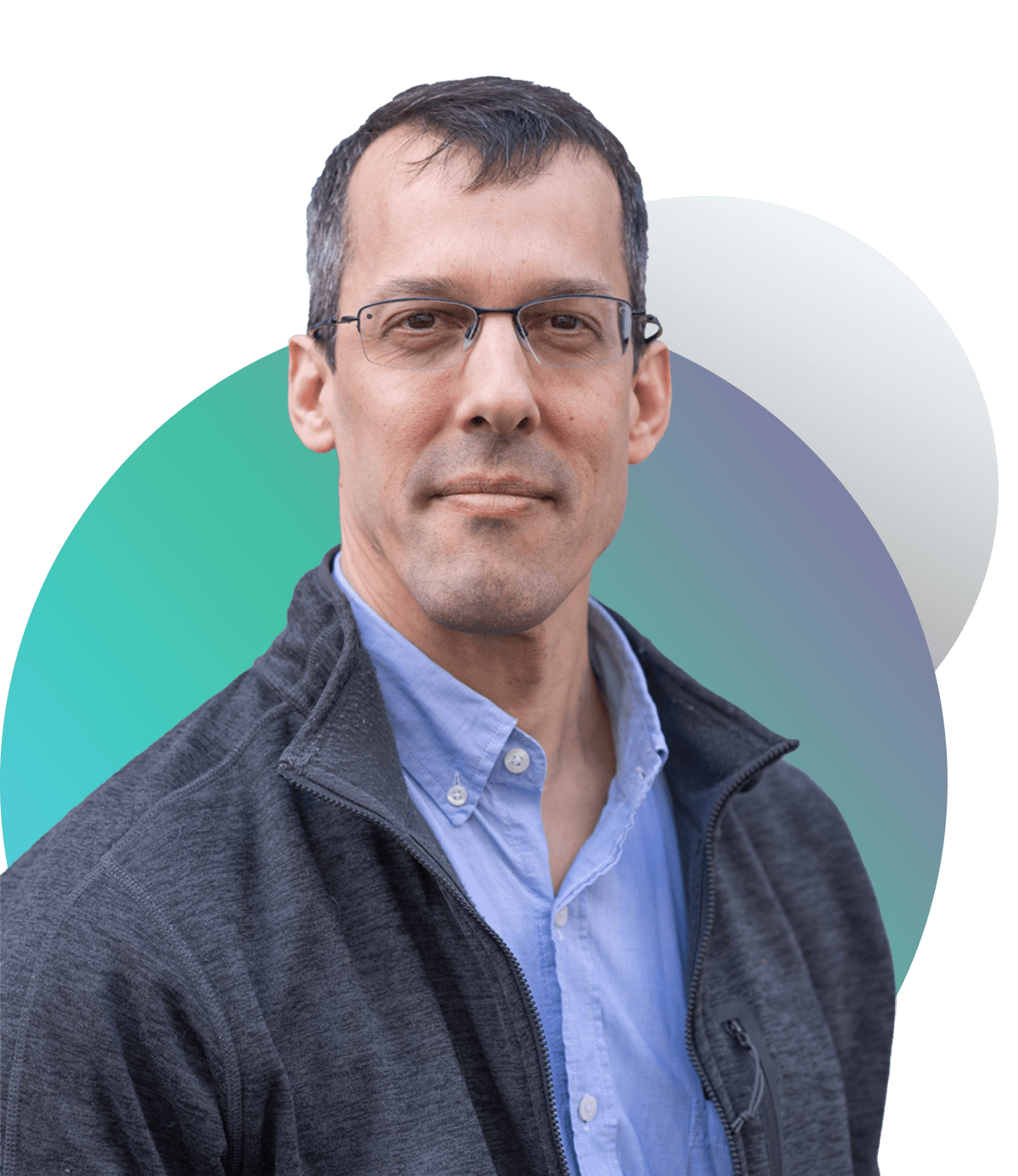
“Humans will have to come to a consensus on how to define consciousness,” Zikopoulos says. “We still don’t have a good definition of what that is.”
“We’re going to find ourselves, very quickly if we haven’t already, where the question isn’t just one of defining the line between technology and biology, but instead needing the help of social scientists and humanists to determine: What does it mean to be human?” says Eric Kolaczyk, a CAS professor of mathematics and statistics and director of BU’s Rafik B. Hariri Institute for Computing and Computational Science & Engineering. “Assistive devices and technologies, if we get them down to the cellular level—what will that mean for what we consider to be human? And how do we channel those questions, societally, in a responsible manner?”
Kolaczyk says the Hariri Institute is set up to facilitate conversations that examine the interface of humans and technology, such as how human tendencies like bias can—and often do—make their way into technologies.
“It’s not a new problem to realize that if you have obtained data in a biased fashion, your analyses will be impacted by that,” he says. “It’s a problem under incredible amounts of scrutiny, ranging from simply having bias exist in a dataset to correcting for that and enabling human-computer interactions that can be as unbiased as possible.”
Engineering Biology
Scientists are also taking the insights gathered from increasingly powerful imaging, sensing, and probing technologies and applying what we’ve learned about the mechanics of tissues, cells, and molecules to develop new biologically based tools.
Imagine, for example, a future where organs could be grown synthetically or manufactured to help address the global shortage of transplantable organs. Or food proteins or carbohydrates could be fabricated for consumption on demand, without the need to grow whole animals or plants.
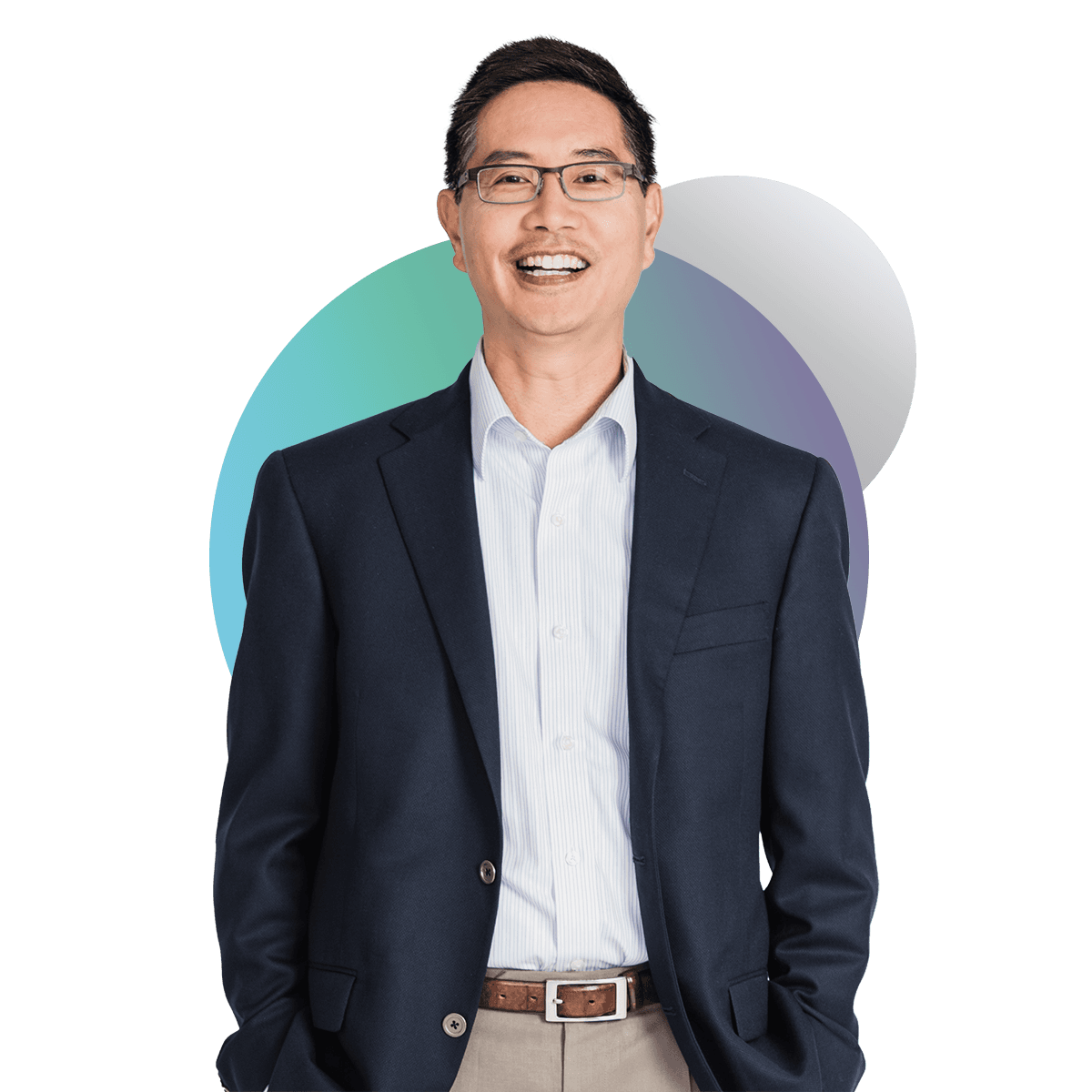
“Until now, biology has mostly been a science of description—like we might think of astronomy—as the field has been focused on trying to figure out how things work,” says Chris Chen, a College of Engineering professor of biomedical engineering and of materials science and engineering, and founding director of BU’s Biological Design Center. “Engineering has always been about trying to understand something well enough to make good use of it…. There’s been this gradual transition from developing tools to understand how things work—molecular biology tools, genetic engineering, etc.—to a mindset of, let’s build these things to figure out what we can change and control in a biological system.”
The idea of “engineering biology” has already come to life in the form of CAR (chimeric antigen receptor) T-cell therapies, which can set genetically engineered immune cells into attack mode on hard-to-treat cancers; with the development of genetically modified plants that can better withstand environmental conditions or weather related to a changing climate; and most recently, and notably, even with the invention of the synthetically derived mRNA used to power Moderna and Pfizer-BioNTech’s COVID-19 vaccines.
“Developments like these have only been possible since the rise of synthetic biology and genetic engineering,” Chen says. “Now that the line has blurred, I think there’s a lot more interest in figuring out what we can control.”
Synthetic biology is an emerging area of research that designs and fabricates new biological parts, devices, and systems, often directly inspired by computer hardware and software, as well as the parts and inner workings of living cells, organisms, and other natural systems.
The possibility is very high that engineered tissues or organs may look very different than the ones we’re born with. Airplanes, which were first inspired by birds, are a good example. Today they don’t look like birds, but they do both have wings.
Over a decade ago, Chen coinvented organs-on-chips technology with collaborators at the Wyss Institute for Biologically Inspired Engineering and Boston Children’s Hospital. Today, organs-on-chips—plastic microchips containing structures and tissues that mimic working units of human organs—are commercially available. They are being used by pharmaceutical companies to test drug compounds with more accuracy than animal-based research models and being developed for a variety of other future uses, including personalized medicine.
And now that it’s possible to mimic units of organs—such as a kidney’s nephron or a lung’s air sac—Chen says the basic stepping-stones are in place for scientists to tackle much more ambitious goals, like engineering functional whole organs.
What might those structures look like? “The possibility is very high that engineered tissues or organs may look very different than the ones we’re born with,” Chen says. “Airplanes, which were first inspired by birds, are a good example. Today they don’t look like birds, but they do both have wings. When it comes to engineering organs, as we start to better understand what matters most to function, we’ll be able to separate out what’s necessary and what’s not in terms of structure and design.
On-Demand Synthetic Biology
“COVID, for better or worse, has shown the world that we are all affected by biology, and our ability to engineer solutions to biological problems quickly is going to be vital in the future,” says Douglas Densmore, an ENG professor of electrical and computer engineering. Biology is essentially nature’s way of programming living things, making it an attractive platform for engineers who see its potential to control the health of people or even entire ecosystems.
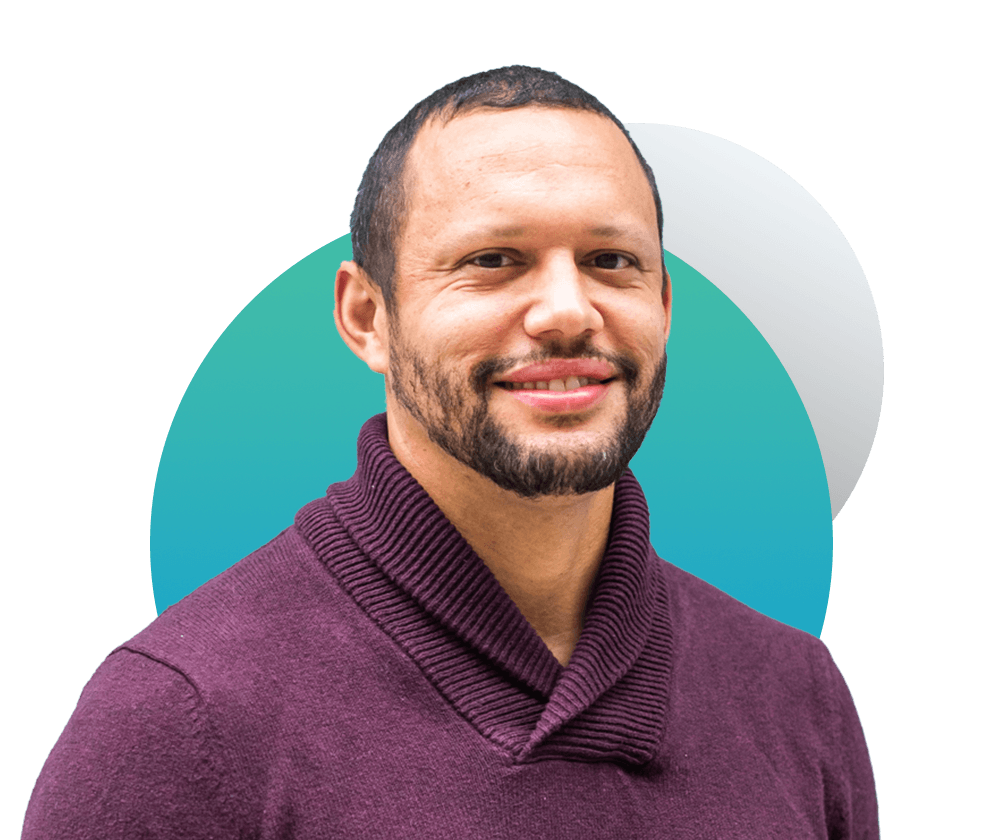
“You and I are the living, breathing, fully realized potential of biology,” Densmore says. “It’s biology making our thoughts and words. It’s biology controlling that we self-assembled into having two arms, two hands. In the 1940s, ’50s, and ’60s, people realized we could take silicon and add electrons to make conductors and computers. Now instead of computers, we can read and write DNA. It’s a new way to encode information.”
Densmore wants to engage young people in the future of synthetic biology; he’s the principal investigator on a three-year, $2.3 million US Department of Defense grant that will provide underserved high school students with a crash course in all things synthetic biology and access to biotechnology companies.
In his lab, he’s working on making biological innovation as approachable as placing a custom-made order. Densmore imagines that someday synthetic biology could be even more widely distributed, perhaps available through yet-to-be-invented machines that could sit inside our homes, as common as printers.
“Biomanufacturing centers could be used on demand to create food, therapeutics, materials, maybe even units of sustainable energy,” Densmore says. “These centers or machines could manufacture engineered bacteria to help fertilize our gardens, cells that look and taste like a sweet potato, or sustainable building materials to patch up leaks in our homes.”
COVID, for better or worse, has shown the world that we are all affected by biology, and our ability to engineer solutions to biological problems quickly is going to be vital in the future.
Allyson Sgro, an ENG assistant professor of biomedical engineering, would likely want to see a machine like that be capable of pumping out on-demand tissues to protect and heal wounds better than modern-day Band-Aids. “Our current wound-healing strategies are imperfect; we can heal from a deep cut, but we’re not great at it,” she says. “Microbial populations have much better ways of restructuring themselves, adapting robustly for survival—I find their coping toolkit unexpectedly inspiring.”
Sgro studies how microbes respond to, and thrive within, changing environments—she’s looking for the biological “algorithms” that they use to sense their environment, process that information, and adapt accordingly. Slime molds, Sgro says, have impressed her with the different ways they create new functions for themselves to solve problems like food shortage or environmental instability.
“Can we create a system in which any person can dynamically respond and ‘fix’ themselves in response to a threat?” Sgro wonders. “Could we someday deliver a liquid treatment to a wound that would dynamically sense the state of the wound, and adapt over time to support wound-healing at every stage of the process? Right now, we have to change treatments each step of the way. If the treatment itself ‘knew’ the best way to treat an infection, it could really be life-changing for patients who live in low-resource settings and who can’t easily get to the doctor multiple times.”
Sgro hopes a similar approach could even be used to develop dynamic cancer treatments, to treat complex infections, or heal myriad other conditions. And the possibilities could extend far beyond human health. She dreams of a day when microbes could be deployed to clean up environmental hazards like oil spills or atmospheric carbon, and then safely self-destruct afterwards.
“I think we’ll have a lot more jobs for living machines in the future,” Sgro says. “Issues like climate change are going to require a dual-pronged approach, where we both change our behaviors and develop new technologies. Living systems already have ways of dealing with carbon dioxide”—just take a look at plants. “More broadly, I think there’s a huge opportunity for growing more dynamic solutions and programming biological systems to sense where threats are in the environment and then dispatching those threats.”
Comments & Discussion
Boston University moderates comments to facilitate an informed, substantive, civil conversation. Abusive, profane, self-promotional, misleading, incoherent or off-topic comments will be rejected. Moderators are staffed during regular business hours (EST) and can only accept comments written in English. Statistics or facts must include a citation or a link to the citation.