A Star Is Born
Unraveling the magnetic mysteries of stellar birth
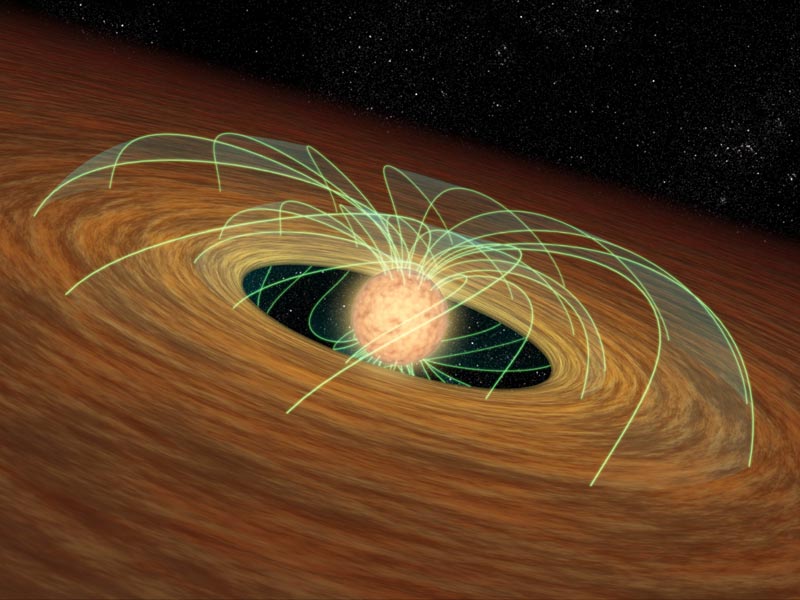
Artist’s conception of a dusty disk orbiting a whirling young star. The green lines represent the magnetic field. Image credit: NASA/JPL-Caltech
How are stars created?
The question has tantalized scientists since the first astronomers turned their telescopes to the sky. But it’s only recently that answers have begun to emerge. Today, we know the basics: a star begins as a huge, swirling cloud of gas and dust that gradually spins into a disk. The spinning disk, which will eventually become a new solar system, gathers a dense ball of matter at the center, with mass flowing from the disk onto the ball in a process called “accretion.” If the ball becomes massive enough to fuse atoms of hydrogen, then a star is born, powered by the nuclear fusion at its core.
That’s the big picture, but the details are hazy. Researchers still don’t know exactly how or why the clouds collapse to form stars, and how it accretes the mass through the disk. But Ian Stephens, a postdoctoral associate at Boston University’s Institute for Astrophysical Research, has brought science one step closer to understanding the process of star formation.
For the first time, Stephens has directly observed the magnetic field in the disk of a baby star—thought to be critical for a star’s birth—and found its shape to be more complex than anyone expected. The discovery, published on October 30, 2014, in Nature, gives new clues—and raises new questions—about how stars are born. And because the baby star Stephens examined will be similar to our sun, his work may shed light on the origins of our solar system.
“The magnetic field in the disk around a star is where planets are formed,” says James Jackson, a College of Arts & Sciences professor of astronomy at BU and Stephens’ advisor. “We wouldn’t be here unless our own sun had done this. This is how we came to be.”
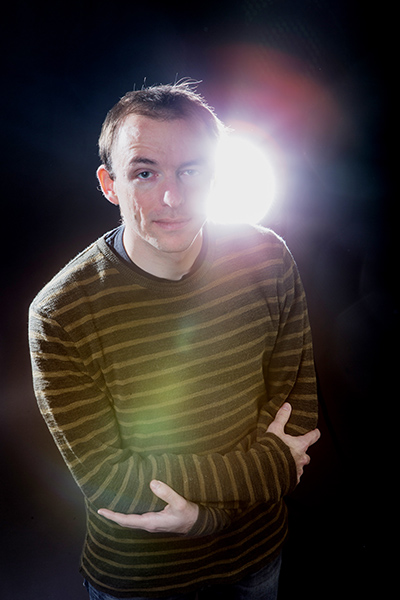
Stephens is investigating one of the biggest puzzles of star formation, what astrophysicists call the “accretion problem.” As clouds of rotating gas and dust collapse, they begin to accrete, or deposit, matter onto a core. But, like a spinning figure skater pulling her arms in tight, the cloud spins faster and faster as it gets smaller and smaller. At some point, the spinning dust cloud seems to violate the laws of physics, leaving astrophysicists scratching their heads.
“The clouds that collapse and form stars are already spinning. But when they collapse into the baby star, the gas would be spinning so fast that, according to the equations, it should actually spin apart,” says Stephens. “But, obviously stars do form, so how does the mass get on the star? That is a big problem.”
Astrophysicists have theorized that magnetic fields may somehow stabilize the spin and help control the accretion through the disk. Other scientists have looked for evidence of these magnetic fields in disks but haven’t been able to find them.
A few years ago, a California telescope called CARMA (the Combined Array for Millimeter-wave Astronomy) got a tool to measure magnetic fields by analyzing the polarized light emitted from the dust. Stephens decided to point the telescope at the disk of a young star called HL Tau, about 450 light years away. And for the first time, he found the predicted magnetic field.
“I’m not the first person to try this, I’m just the first person to try this successfully,” says Stephens. “I did what I thought was the most obvious thing—I pointed the telescope at the brightest disk possible. Other people have pointed it at the biggest disk or the closest disk, but I pointed it at the brightest. And that worked.”
But there was a surprise. The shape of the magnetic field was more complicated than anyone expected. Some models had predicted it would be circular or “toroidal” and others suggested it would be moving toward the disk’s poles, or “poloidal.” Instead, it was neither.
“This is quite a find,” says Jackson. “If we can find out how the magnetic fields form around the disk, we can figure out if they’re as important as the theorists predict.”
Stephens isn’t quite sure what the finding means for the process of star formation, except that it’s likely more complicated than anyone thought. “To me, the finding says that the models we’re using are too simplified,” says Stephens. “At the same time, for the models to really have constraints, we really need more observations.”
To that end, Stephens is planning another round of observation for 2015, at the $1.1 billion Atacama Large Millimeter/submillimeter Array (ALMA) telescope in Chile, the best telescope in the world for making the long-wavelength measurements that interest Stephens. He’ll be collecting data for HL Tau again, but this time in much greater detail. He’s not sure what he’ll find, but hopes it will add at least one more piece to the puzzle of star formation.
“I hope the new observations do answer some of these questions, but so often, observations just lead to more questions. How did our sun form? How did it form the solar system, and how did it form Earth?” asks Stephens. “Astronomy is like looking back in time.”
Comments & Discussion
Boston University moderates comments to facilitate an informed, substantive, civil conversation. Abusive, profane, self-promotional, misleading, incoherent or off-topic comments will be rejected. Moderators are staffed during regular business hours (EST) and can only accept comments written in English. Statistics or facts must include a citation or a link to the citation.