2024 Kilachand Fund Awards Boost Projects Building Antivirus Platform, Improving Brain Imaging, and Fighting Antibiotic Resistance
Interdisciplinary research fund was established to tackle biggest challenges in the life sciences
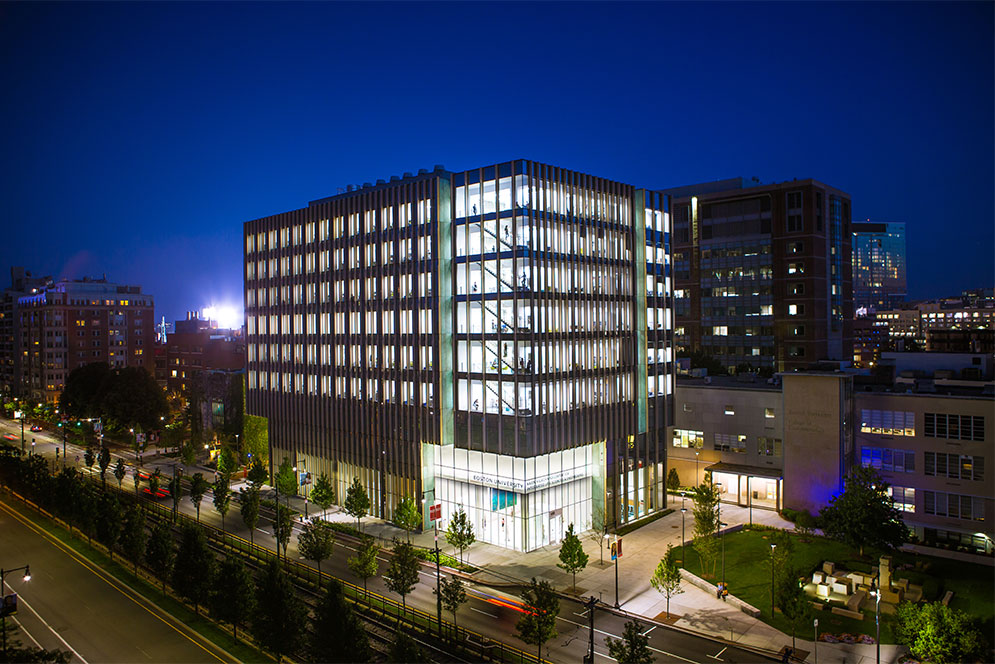
Photo by Janice Checchio
2024 Kilachand Fund Awards Boost Projects Building Antivirus Platform, Improving Brain Imaging, and Fighting Antibiotic Resistance
Interdisciplinary research fund was established to tackle biggest challenges in the life sciences
Messenger RNA (or mRNA) vaccines showed their potential as critical virus response tools during the COVID-19 pandemic. But mRNA vaccines come with limitations, including a lack of immediate antiviral immunity and high dosage requirements. Another antivirus tool, antibodies, can provide immediate protection against viral infection (from Ebola, for example), but are limited by slow manufacturing processes and logistical access challenges.
What if we could find a way to bring mRNA vaccines and antibodies together, so each retains their benefits while reducing their limitations? A new Boston University project aims to do just that.
“We’re seeking to mitigate the limitations of mRNA vaccines and antibodies through a single system,” says Florian Douam, a BU Chobanian & Avedisian School of Medicine assistant professor of virology, immunology, and microbiology. “Our project proposes to synergize three technologies recently developed at Boston University: a self-amplifying RNA (saRNA), an automated nanobody screening platform, and computational tools for predicting RNA function. When combined together, these technologies solve the design, manufacturing, and delivery challenges of antibodies.”
The project is one of three to win a 2024 Rajen Kilachand Fund for Integrated Life Sciences & Engineering award. The other two Kilachand Fund award–winning projects this year are pursuing ways to improve neuroimaging of the brain and attack antibiotic resistance.
According to Douam, mRNA is used in vaccines to spark the development of proteins that teach the body how to fight future infections, and the lab-made saRNA is like a supercharged version—causing more protein production, even at ultralow doses. The nanobody screening platform can allow scientists to discover virus-battling antibodies quickly, while the computational technology helps optimize RNA structure and maximize antibody production. Together, they could become a powerful and versatile tool that could be adapted as a first line of defense against a future pandemic.
It’s the kind of interdisciplinary project the Kilachand Fund awards were designed to lift up. BU trustee Rajen Kilachand (Questrom’74, Hon.’14) established the award fund in 2017 with the goal of spurring solutions to some of the biggest challenges in the life sciences.
“As we confront the toughest challenges in life sciences and engineering—from heart disease to cancer to neurodegenerative disorders—convergent research teams fueled by strategic University commitments have proven to be powerful engines for advancing fundamental discoveries and catalyzing transformational progress,” says Thomas Bifano, BU’s vice president and associate provost ad interim for research.
Improved Defenses Against Viruses
About a year ago, a lab team led by BU biomedical engineers Wilson Wong and Mark Grinstaff discovered a novel way to modify saRNA, a breakthrough they’ve since used to create a more effective COVID-19 vaccine in collaboration with Douam. Using that capability along with the nanobody discovery platform, developed by Ahmad (Mo) Khalil, a BU College of Engineering professor of biomedical engineering, could offer what Douam calls “a novel pipeline to discover, develop, and implement preventive and therapeutic tools against viral infection and viral pandemic.” The computational technology was designed by Alexander A. Green, an ENG associate professor of biomedical engineering. The Kilachand Fund award’s support will allow the team to build a proof of concept.
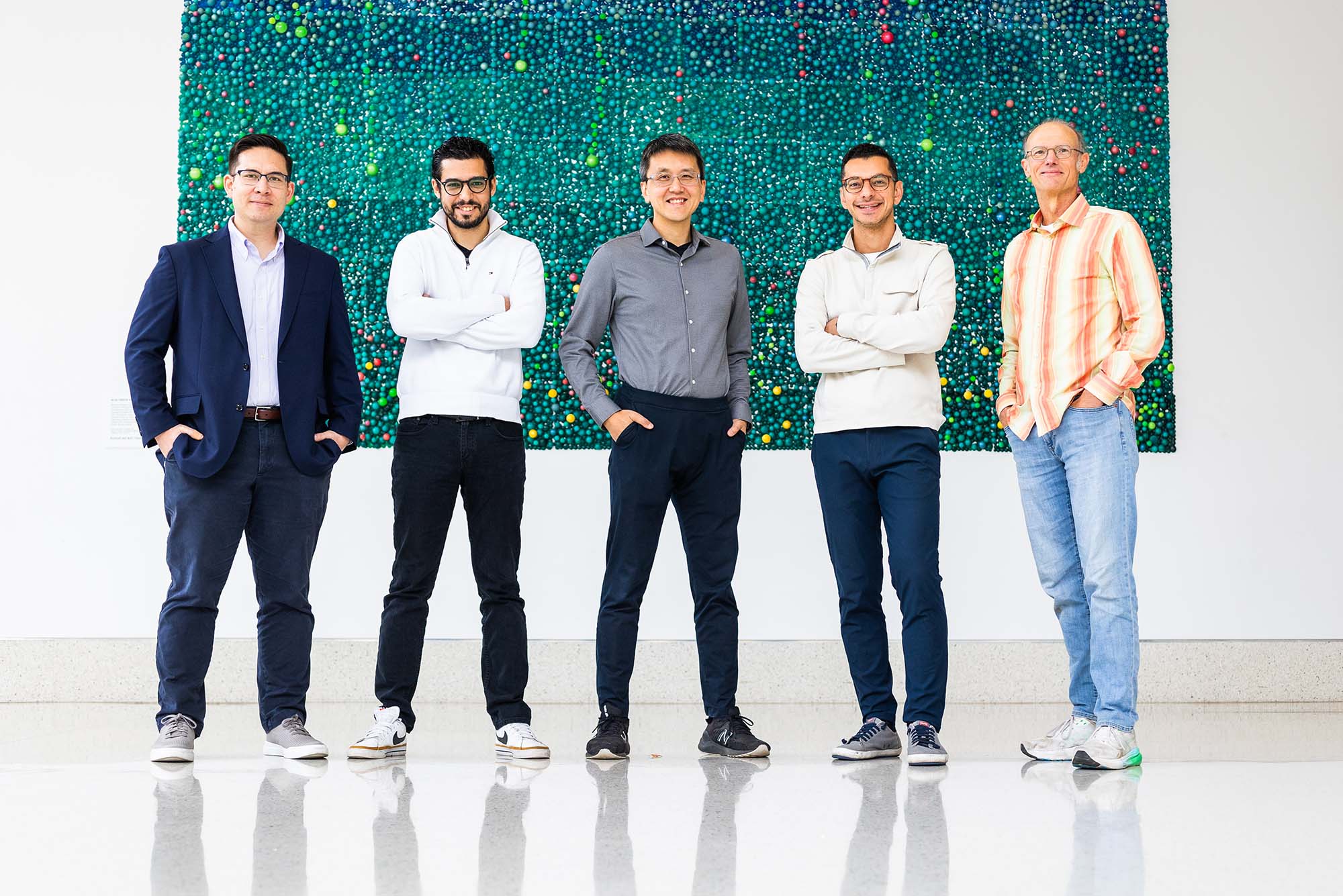
By encoding the genetic sequence of antiviral antibodies into the saRNA, says Douam, who’s also a core investigator at BU’s National Emerging Infectious Diseases Laboratories (NEIDL), “we can confer immediate protection against any viral pathogen; you’re going to be protected right away, a big advantage over mRNA.” And because it only requires a low dosage and can be injected “into the arm of patients, directly producing the antibody without an inflammatory response,” he says, the technology would be “easily scalable, cost-effective, and rapidly deployable in the field.”
Douam is leading the project with Wong; Green, Grinstaff, and Khalil are coinvestigators.
Transforming Our Understanding of the Brain
Functional magnetic resonance imaging, or fMRI, provides a noninvasive way to study human brain function. The neuroimaging technique illuminates regions of the brain involved in tasks such as language development and reveals differences in brain activity patterns in conditions like depression, schizophrenia, ADHD, and autism. This understanding of the brain’s underlying neural mechanisms has enabled the development of new treatments—but we may have been misreading brain scans for years, according to BU researchers.
By studying the brains of mice, they’ve found the vascular response—how blood vessels react—to neural activity, called neurovascular coupling (or NVC), is not constant in time, which has long been assumed when interpreting neuroimaging results. Their results also suggest neuromodulator signaling—a physiological process that modifies and regulates communication between neurons—might be altering NVC.
With backing from the Kilachand Fund award, the project team aims to expand their research, eventually moving to examine these processes in humans. Besides driving improved interpretation of neuroimaging results, the project could also enable new approaches for investigating and treating conditions as diverse as Alzheimer’s disease and migraines.
Because fMRI measures the hemodynamic (blood flow) response to brain activity, “it’s not measuring neural activity directly,” says David Boas, an ENG professor of biomedical engineering. “We need to know the relationship between neural activity and the vascular response in order to interpret fMRI. If the vascular response is changing in time, that’s going to potentially alter how we interpret many [neuroimaging] findings.”
The project’s work is being conducted by principal investigators Boas, Emily Stephen, a College of Arts & Sciences assistant professor of statistical neuroscience, and Mike Esterman, a Chobanian & Avedisian School of Medicine associate professor of psychiatry.
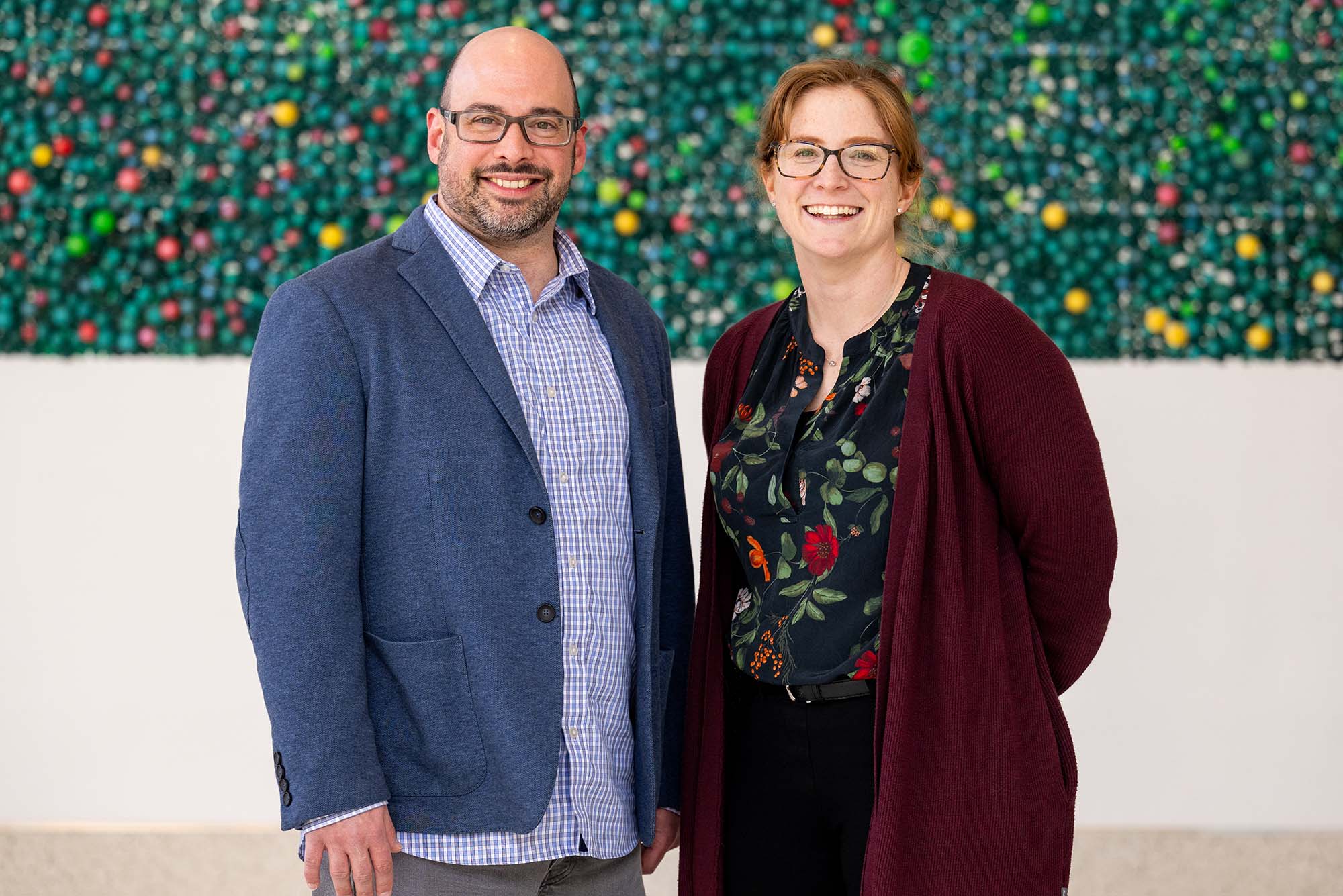
Boas says their work could unlock new insights into the release of neuromodulators, which are brain chemicals like dopamine, serotonin, and norepinephrine that alter the activity of neurons in the nervous system.
That deeper understanding of the role of neuromodulatory signaling dynamics could change what we know about various neurological disorders. “Just take norepinephrine, which we have already found in mice to alter NVC,” says Boas. “Norepinephrine signaling is known to be altered in Alzheimer’s disease, depression, and chronic pain and migraine. So we could actually get more insight into the dynamics of norepinephrine, which could open up the potential for new discoveries.”
The project team will extend their investigation in mice to understand if other neuromodulators—including acetylcholine, serotonin, and dopamine—also alter NVC.
“This will expand the clinical relevance of our findings to include Parkinson’s disease, schizophrenia, Huntington’s disease, and anxiety disorders,” says Boas. “The Kilachand Fund award really helps us bring together the multidisciplinary team needed to solidify the foundations of the work we’ve done.”
Overcoming Antibiotic Resistance
When bacteria become resistant to available antibiotics, infections that were once easily treatable become difficult, potentially impossible, to treat. That means prolonged illnesses and complications, as well as higher mortality rates. A 2022 analysis published in The Lancet reported over six million deaths globally in 2019 in which antibiotic resistance was a direct or contributing factor.
Mary Dunlop, an ENG associate professor of biomedical engineering, describes increasing antibiotic resistance as “a massive global health problem,” explaining that “the speed at which drugs are clinically approved is being dramatically outpaced by bacteria’s ability to acquire resistance.”
Bacteria can become resistant to antibiotics in two ways. The first is through mutations that modify the drug’s target, either a protein or an enzyme that a drug affects. The second (and primary) way is through a process called horizontal gene transfer, which allows bacteria to pass genetic materials (including resistance genes) between cells.
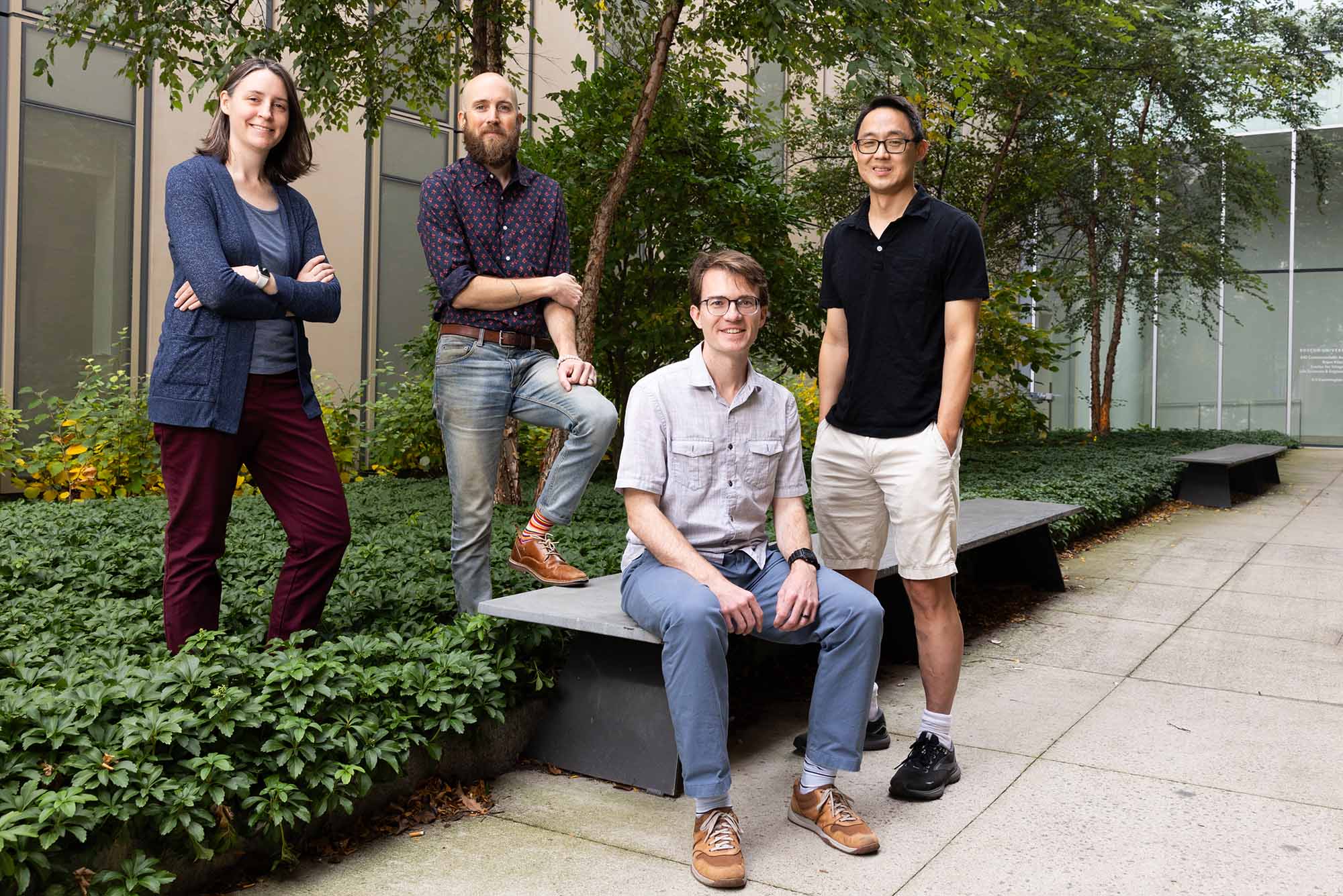
“Our project focuses on horizontal gene transfer and the single-cell level events that are involved in making an individual bacterium transition from being drug-susceptible to drug-resistant,” says Dunlop.
Led by principal investigators Dunlop, Douglas Holmes, an ENG associate professor of mechanical engineering, Joseph Larkin, a CAS assistant professor of biology and physics, and Harold Park, an ENG professor of mechanical engineering, the project team proposes using light to trigger the horizontal gene transfer event so that it can be observed in real time. “We can initiate horizontal gene transfer in one location, and then watch the spread within the community,” says Dunlop. The technique is a form of optogenetics, which uses light to influence and control cells.
Existing research on horizontal gene transfer has looked at the population level—analyzing the characteristics of a group of cells as a whole to observe average behavior or trends—rather than the individual cell-to-cell level. But Dunlop says “these processes are fundamentally happening between cells, and understanding cell-to-cell transfer could tell us a lot about how to counteract the spread of antibiotic resistance via horizontal gene transfer.”
Doing these single-cell level studies, especially using optogenetics to control horizontal gene transfer, is innovative. “I can’t think of anybody else working on it, which means there’s high potential here, and also inherent risk,” says Dunlop. “This award enables our team to develop these ideas and generate proof of principle computational models and experimental tools that will allow us to build a foundation and extend the work.”
Comments & Discussion
Boston University moderates comments to facilitate an informed, substantive, civil conversation. Abusive, profane, self-promotional, misleading, incoherent or off-topic comments will be rejected. Moderators are staffed during regular business hours (EST) and can only accept comments written in English. Statistics or facts must include a citation or a link to the citation.