From Ant Brains, Seeking New Lessons about Human Behavior and Society
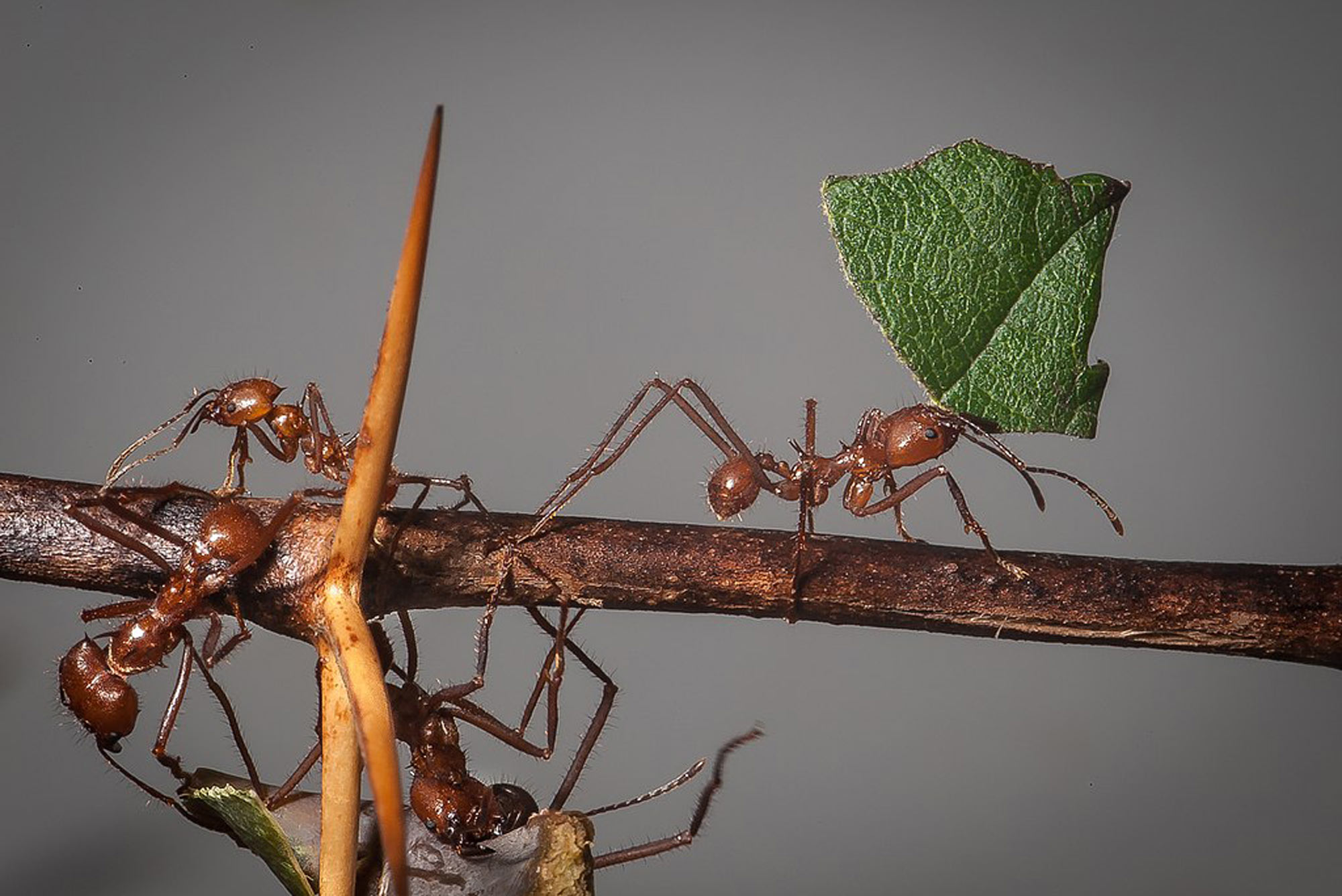
Photo by Pjt56/Wikimedia Commons
From Ant Brains, Seeking New Lessons about Human Behavior and Society
$1.5 million in new grants will support BU biologists researching the links between brain evolution, socialization, diet, genes, and more
What can ants tell you about the human brain—and society? A whole lot, it turns out. Ants, like humans, are highly social creatures, meaning that their brains have developed special structures and functions to enable cooperation and connection with their community. Some types of ants even work together to cultivate and harvest mushrooms for food.
So, looking at these agricultural ant “farmers” and other species, Boston University biologist James Traniello is studying the complexities of ant brains and how brain features have evolved to correspond with behavior and sociality.
For his efforts, he and his collaborators have recently been awarded three new grants totaling nearly $1.5 million, which will fund new studies looking at ant brain evolution, structure, function, gene expression, diet, metabolism, socialization, and more. Although the coronavirus pandemic has sidelined much of their lab work for months, the team is slowly phasing back toward in-lab research. Traniello, however, admits he’s suffering from “lab withdrawal” despite the fact that his team meets weekly over Zoom.
“I sorely miss being at 5 Cummington Way with everyone in person,” he says. But, with BU labs ramping their activity back up in line with the University’s reopening plan, Traniello and his team will soon be donning their lab coats to study ants with exciting new research questions in mind.
The Brink reached out to Traniello and the members of his lab who will be leveraging the new funds—awarded from the National Science Foundation, the BU Genome Science Institute, and Fondo Nacional de Desarrollo Científico, Tecnológico y de Innovación Tecnológica—to ask them about their research, what exactly they hope to learn about ants (and other animals and maybe even humans), and, as the coronavirus pandemic continues to spread, whether humans can learn any lessons from disease-fighting ants. (Spoiler alert—the answer is yes.)
Here’s what Traniello and his team—including former doctoral student Franne Kamhi, current PhD candidates Zach Coto, Isabella Muratore, Frank Azorsa, and undergraduate researchers Mimi Kay and Faraz Zaidi—had to say.
Q&A
With the Traniello Lab
The Brink: First, a chicken or the egg type of question. Do brains evolve to enable complex social relationships, or is the opposite true, do complex social relationships and societies influence brain size and structure?
Traniello Lab: In vertebrates, the relationship between social complexity and brain size and structure is thought to be a coevolutionary “arms race” of [natural] selection favoring personal gain. For example, the complexities of social life in primates that live in large groups require cognitive abilities to respond adaptively to different social situations. If enhanced social cognition requires greater information-processing ability, then the result may be an increase in brain size. Larger brains, in turn, may enable more complex behavior, in turn selecting for greater processing ability.
But in social insects like ants, brain evolution is related to the sterility of workers and the fitness of the colony rather than the individual. Cognitive challenges that influence worker ant behavior and consequently their brain size and structure are associated with behavioral specializations—division of labor, which involves the evolution of workers that vary in size and the tasks they perform, is important. Some workers may have more diverse and demanding behavioral repertoires associated with foraging, whereas others may be specialized to defend the colony, grind seeds, or serve as a living door to a nest. We expect the brains of workers with either broad or extremely narrow task repertories to differ in size and neuroarchitecture to fit their social roles.
Where does brain metabolism fit into this picture? How does it influence brain complexity and the social nature of a species?
Larger brains should cost more energetically to develop and operate. Brains are a small fraction of the total mass of an animal, but they use a disproportionate amount of energy. For example, human brains weigh only about 3 pounds, but use about 20 percent of our energy. Brains are also metabolically selfish, potentially capable of demanding energy dedicated to other organs.
If evolution favors larger brain size, how does this impact energy demands? Social insect brains are minute and physiologically different from vertebrate brains. Franne Kamhi, who earned her PhD doing research in the Traniello lab, showed that some socially complex ants have larger brains, but use less energy to operate them. This relationship between social complexity, brain size, and metabolism isn’t well understood, and is the focus of work now led by Traniello lab member Zach Coto, who is pursuing a doctoral degree.
What can studying ants teach us about human brains and societies? Why are ants such an important research model?
How brain structure and metabolism have been impacted by social life is an important unresolved question across all species. Our approach is to answer it by broadening conceptual perspectives of brain evolution using ants as models, and integrating evolutionary biology and neuroscience.
Like humans, ants are known for their extraordinary collective intelligence. Cooperating groups are better at problem-solving than individuals in both human and ant societies, so we can ask how emergent cognition affects brain evolution, including metabolism.
There is also the idea from complex systems theory that greater social complexity is derived from individual simplicity, but how group-level cognition influences brain size, structure, and metabolism isn’t well studied. So, ants provide opportunities to address questions that are also significant in human evolution.
Dietary and cultural change are thought to be associated with the formation of larger and more stable social groups, changing cognitive demands of group members that may be reflected in brain size, organization, and genetics. It’s intriguing that human brain size appears to have decreased since roughly 10,000 years ago, around the time that agriculture originated.
It’s difficult to answer the question of how sociality and collective problem-solving influenced human brain evolution, because the human fossil record limits analysis. However, there are linkages of social complexity, cognition, and changes in brain size and body size in agrarian ants that give insight into what’s possible in nature under broadly similar circumstances.
How will single-cell RNA sequencing help you understand the biological mechanisms that give way to complex brain structures? What types of molecular signals are you searching for?
We want to understand how gene expression underpins brain structure and behavior. Traditional RNA sequencing studies are often limited by assuming a cell population is homogeneous (or all exactly alike) in gene expression, but even though all cells share the same genome, only a subset of genes are [activated] within individual cells. This may vary across different types of cells and among very similar cells or populations of cells in different areas of the brain. This variation is thought to be important in cell development and function, and in the agricultural roles that ants play, but we can’t characterize it using whole-brain RNA sequencing studies because those only reflect [a melting pot of genes], or the average expression levels of individual genes across the entire brain.
By using single-cell RNA sequencing, however, we can see whether certain genes are more or less active in very specific parts of the brain. We predict that patterns of differences in gene expression across the brain—with some genes being more or less active in a certain part of the brain than another—will reflect how metabolic and behavioral functions are controlled by genes located in brain subregions. Sean Mullen, a BU College of Arts & Sciences associate professor of biology, and Isabella Muratore, a PhD candidate in the Traniello lab, are exploring this.
Why did you choose to study Atta cephalotes for this purpose? What about fungus-growing ants makes them more interesting for study than more common ant species?
Fungus-growing ants produce their own food supply, a crop of mushrooms. Only ants, termites, and humans are ultrasocial—meaning they practice agriculture and have full-time division of labor.
Atta cephalotes is remarkably varied in its appearance; workers range in size from tiny minims (roughly a half-millimeter across the head) to majors (more than 3.5 millimeters) and can be divided into groups called subcastes according to their size and the tasks they perform. Their brains differ structurally, especially in a compartment called the mushroom body, which is deeply related to the vertebrate cortex. This is the worker brain’s center of learning and memory, and it’s proportionally larger in the subcaste that has the most complex behavior.
We hypothesize that differences in brain structure like this and cell-specific patterns of gene expression underpin agricultural division of labor in this ant by influencing brain makeup and behavior in subcastes.
Fungus-growing ants offer great opportunities to understand brain evolution because they’re diverse in social organization. Some species have small colonies with workers of roughly equal size that perform the whole array of tasks needed to produce a crop, but in advanced species that have large colonies, farming is on an industrial scale, and is accomplished by the evolution of different-sized workers that vary in their behavior. So, within a clade of ants, there is great diversity in social complexity, which we can leverage to explore our research questions about the links between socialization and brain evolution.
Other ants even further extend these research opportunities. A group of ants called poneroids are known for their predatory habits, and are considered to be the most primitive of ants. Like fungus-growing ants, they are sociobiologically and ecologically diverse, but the workers in this group are active predators, and among different species they show striking differences in diet, colony size, body size, worker appearance, and division of labor. Some species of poneroid ants prey only on termites, whereas others are generalists.
Poneroid ants vary in body size from 1.4 millimeters to the “huge” 3.6-centimeter-long Dinoponera gigantea, and colony size can be fewer than 10 or more than 10,000 individuals. Differences in diet specialization, feeding behavior, and social organization are likely associated with cognitive adaptations related to their diets and sociality that influence brain size, structure, and metabolism, making them an ideal group to test theories of brain evolution. Frank Azorsa, a PhD candidate in the Traniello lab, is researching poneroids for his dissertation project.
How has the COVID-19 pandemic impacted your research, or that of the doctoral students in your lab?
We had to close our lab in March, and we’ve just recently started to reopen. The doctoral students and undergraduates working in the lab have been great coping with the change. PhD candidates Zach Coto and Isabella Muratore have been maintaining the ant cultures and attending to other essential lab work since March.
Frank Azorsa, who received funding to study the effect of diet on brain evolution in predatory ants, is currently in Peru, unable to make ant collection trips and unable to return to Boston because airports are closed. Hopefully, he’ll be able to collect ants soon and return to the lab at BU.
Undergraduate researchers Mimi Kay and Faraz Zaidi have been able to continue their work remotely.
Are there any interesting parallels you see between ants—and how they might overcome attacks from invasive, parasitic species—and the challenge currently facing humans and other COVID-susceptible species?
Social insects like ants don’t debate the need to practice behaviors that prevent disease spread or contest vaccination. And unlike humans, they don’t need strong leadership and centralized control to prevent pandemics.
Ants live in environments rich in pathogens and parasites and form colonies of potentially millions of individuals that live densely packed inside nests. One of their oldest and most important adaptations to control infection is the metapleural gland, found in the abdomen, which produces antibiotics. Each ant is a pharmacist, secreting prophylactic chemicals and distributing them throughout the colony by grooming themselves, their nestmates, and applying the antibiotic secretions to the entire brood.
In addition, fungus-growing ants coexist with a bacteria that produces antibiotics to control other alien fungi that can invade colonies and kill the mushrooms they are farming for food.
In general, social insects communicate about the presence of pathogens and generate signals that cause individuals to disperse, lowering the risk of contagion. They can “run a fever,” increasing nest temperature to thermally kill pathogens. Also, infected worker ants will physically distance themselves from uninfected nestmates, voluntarily quarantining, and leave the colony before they die.
Our research even shows that some species have social immunity—workers with immunity are able to enhance disease resistance in the colony as a whole, perhaps by inoculating nestmates or distributing immune promoters.
Responses were edited for length and clarity.
Comments & Discussion
Boston University moderates comments to facilitate an informed, substantive, civil conversation. Abusive, profane, self-promotional, misleading, incoherent or off-topic comments will be rejected. Moderators are staffed during regular business hours (EST) and can only accept comments written in English. Statistics or facts must include a citation or a link to the citation.