Could Autism, Depression Be Rooted in Our Brain’s Ability to Pay Attention?
Could Autism, Depression Be Rooted in Our Brain’s Ability to Pay Attention?
BU neuroscientist is developing a simulated human brain to test his theory

Are you able to focus only on the words you’re reading right now and block out all other distractions and thoughts? If so, neuroscientist Basilis Zikopoulos wants to understand how your brain can do that.
He’s studying the attention filter of the human brain, which is at the heart of why we can focus—or why we can’t. And he’s got good reason to spend so much of his time thinking about how our brains pay attention. He has a hunch that autism, schizophrenia, depression, anxiety, and sleep disorders might all stem from the same root problem: disruptions in a part of the brain that he calls the “attentional searchlight.” To test his theory, he and other Boston University researchers are building a lifelike computer model of a human brain, which they will subject to the same cognitive tests used to assess the brain activity of living people.
“Any kind of information that reaches your brain, whether it be thoughts, motives, sensations from the internal state of your body, or things you notice about your environment, has to pass through a structure called the thalamus,” says Zikopoulos, a BU Sargent College of Health & Rehabilitation Sciences assistant professor of health sciences. “As this information reaches the thalamus, it will either be routed into the cortex so that you become conscious about the information or it will be filtered out” and kept in our subconscious.
Determining what’s destined to be sent to our conscious or subconscious is the function of a smaller bit of brain inside the thalamus, and that’s the piece Zikopoulos is most fascinated by. “The thalamic reticular nucleus, or TRN as we call it, acts like a filter…determining which information can go through to consciousness and which information doesn’t reach that level,” Zikopoulos says. “It’s so important—it’s the ‘attentional searchlight’ of the brain that sorts out what we need to pay attention to and what we don’t.”
How the attentional searchlight takes its cues
The TRN plays a central role in the brain networks that determine if we are awake or sleeping—which is why it can be hard to sleep when we’re consumed by thoughts about a new love interest, or a breakup, or a family member’s illness, or a big promotion at work. It’s also why our attention is so easily captivated when we hear threatening noises or see looks of fear or anger on others. That’s the TRN in action, taking its cues from a brain structure known as the amygdala, the emotion regulation center.
The amygdala is so strongly connected to the TRN that “it’s practically yelling at it,” Zikopoulos says. “It explains a lot about why you pay attention to something that’s emotionally significant.”
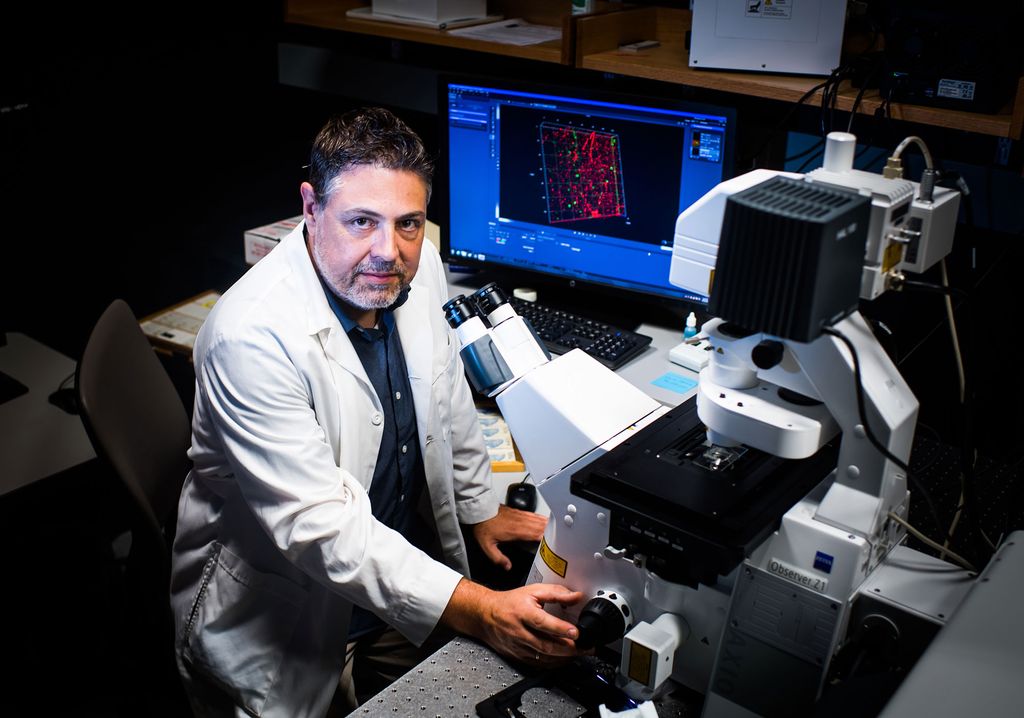
Despite how hard it can be to think of anything else when we’re focused on an emotionally charged thought, nature gave our brain an override mechanism that can (hopefully) direct our attention elsewhere.
The ability to drive our attention toward a specific task, such as I want to focus on my work and ignore other things, Zikopoulos says, depends largely on the executive functioning part of the brain, the prefrontal cortex, which in humans has greatly evolved and expanded compared with other animals. It determines our individual personalities, cognitive skills…and it’s directly connected to and can control the TRN. But the enormous flexibility in our ability to control our own attention and behavior comes at a high cost.
“You don’t see animals that have autism or schizophrenia,” Zikopoulos says. “But humans are more vulnerable because we have more complex brain networks that take longer to develop. Therefore more things can go wrong.” That idea is at the core of his working hypothesis that emotional and psychiatric disorders stem, in many cases, from abnormal links between the TRN and other parts of the brain.
Blazing attention’s trail—in monkeys
“In autism, for example, we see extremely focused attention on one thing, with difficulty switching to another task. In schizophrenia, we have the exact opposite,” he says. “In depression, people focus their attention on negative stimuli and they can’t snap out of it. At the core of all these disorders, we have a problem with attentional networks.”
The TRN is such a new topic of interest for scientists that Zikopoulos, with the support of the National Institute of Mental Health, is now trailblazing the first map of how it is organized and works in humans. As one of the first people to plot the path of TRN networks in monkey brains, he has a good head start.
Together, Zikopoulos and colleague Helen Barbas, a Sargent College professor of health sciences, used neural “tracers” that allowed them to chart the TRN networks in the brains of monkeys. The tracers are designed to be surgically injected into the brain, at which point they begin to be transported throughout all the connections between the brain’s neurons. As they travel, they leave a chemical trail behind them that reveals the brain’s neural networks. Through careful dissection and high-resolution imaging, Zikopoulos and Barbas precisely mapped the three-dimensional networks of TRN connections zigzagging toward other areas of the monkeys’ brains.
“Having done these studies in monkeys, we have identified specific features of the TRN network and how it connects to other areas of the brain,” like the amygdala and the prefrontal cortex, Zikopoulos says. “We think that defining these features in humans could allow us to distinguish between the brains of neurotypical individuals and of people with attention-related disorders.” Such a feat could unlock entirely new insights into why we are who we are, and just how varied the wiring of our brains might be.

One hundred human brains, frozen in time
Born in Greece, Zikopoulos began his undergraduate career with the idea that he wanted to be a marine biologist “like Jacques Cousteau.” But once he got underway on that course of study, he found that the research questions he was asking about the marine ecosystem weren’t as exciting as questions he wanted to answer about how the brains of marine animals had adapted their behavior to aquatic life. He also admits, with a laugh, that he didn’t enjoy cleaning the boats at the marine institute where he was studying.
Zikopoulos’ attention turned to the brain itself. “I went into a neurobiology lab as a second-year undergraduate student and I’ve been there ever since. I have studied the brains of every species you can imagine. Fish, chicken, quail…” He explains that animals that grow inside eggs are good models for studying development, because they are developing outside the body so that their embryos can be easily observed via microscope.
Then after working his way up to studying mammals like rats and mice, he eventually moved on to study monkey and human brains.
Now, he starts every day asking himself what he will discover today: “What makes us special? How are we different from other animals? Why do we have enormous behavioral flexibility?”
To find out those answers, Zikopoulos doesn’t plan on putting the neural tracers to work in living humans (who would then need to donate their brains after death). Based on the data he collected from monkeys, however, he doesn’t have to.
“I already have a whole lab full of donated human brains,” he says. “The brains—nearly 100 in total—come from people ranging between 3 years old and 80 years old…some of them are neurotypical brains, and others come from people with autism, schizophrenia, or depression. We preserve them in special liquids so that the tissue quality remains high for a very long time as we study regions of interest and label brain sections with different markers.” Many of the brain sections in the lab were cryopreserved shortly after their donors died, so that Zikopoulos and his lab members can go in and trace the neural connections as they existed when the donor was still alive.
Using the monkey data and combining it with their observations of human brain specimens, his team plans to collaborate with computational neuroscientist Arash Yazdanbakhsh, a BU College of Arts & Sciences research assistant professor of psychological and brain sciences, to enter all the information into a computer model, which will allow them to create a digital brain. Then, Zikopoulos and Yazdanbakhsh, who are both researchers in BU’s Center for Systems Neuroscience, will ask the artificial neural network—a digital version of a human brain—to complete cognitive tasks similar to those used to assess living people with neurotypical or disordered brains.
“Once we can look at all the data in a computer simulation of a human brain, then we will be able to start disrupting the model and see how it responds in comparison to people that we know have sleep disorders, autism, schizophrenia, depression, etc.,” he says. “It will be an extremely complex computer model for us to build…. We’ve been working on this problem for quite some time.”
Zikopoulos says there are enough questions about the TRN for him to study it forever. He’s been working on unraveling its mysteries for the last fifteen years, and only now feels as though he’s amassed enough pieces of data to start seeing the total picture. “There’s a lot of information and we have no idea what we will eventually find. But we now have some pretty good guesses.”
Comments & Discussion
Boston University moderates comments to facilitate an informed, substantive, civil conversation. Abusive, profane, self-promotional, misleading, incoherent or off-topic comments will be rejected. Moderators are staffed during regular business hours (EST) and can only accept comments written in English. Statistics or facts must include a citation or a link to the citation.