The Atom Smasher
Physicist Tulika Bose talks about smashing protons to find new physics
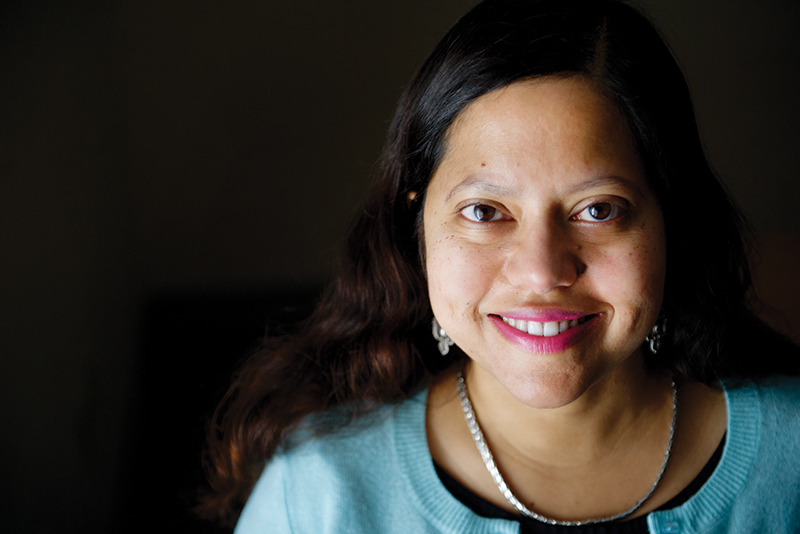
Tulika Bose, trigger coordinator for the Compact Muon Solenoid experiment at the Large Hadron Collider in Switzerland. “Trigger meetings are dramatic places,” she says. Photo by Jackie Ricciardi
Tulika Bose is a science detective. A trigger-happy, atom-smashing, big question-asking physicist, who spends her days sifting though scraps of proton collisions for clues about the universe.
Bose, an assistant professor of physics at Boston University’s College of Arts & Sciences, works at the Large Hadron Collider (LHC) in Switzerland, one of the most powerful particle accelerators ever built. The LHC smashes protons together 40 million times a second, and physicists like Bose sort through the wreckage. Bose is looking for particles (or their remains) that will substantiate (or dismiss) new theories that solve mysteries about the Big Bang and the nature of matter.
In September 2014, Bose was appointed trigger coordinator for the Compact Muon Solenoid (or CMS) experiment at the LHC. She spoke with BU Research about what all those words mean, and why we should bother looking for “new physics” when the old physics seems to work just fine.
BU Research: You were recently appointed the “trigger coordinator” for something called the CMS experiment. Can you explain what that is?
Bose: Sure! At the LHC, we collide protons with protons to see what comes out of the collision, trying to find new particles and new physics. There are four major experiments and CMS is one of them. The name stands for Compact Muon Solenoid—a solenoid is a type of magnet, hence “solenoid” in the name. It’s a particle detector.
How big is it?
15 meters high (that’s about 50 feet) and 15 meters wide. A person next to it looks like a tiny little person.
Then why is it called “compact”? It doesn’t seem very compact!
Very good question. It’s a relative word, because it’s compact in comparison to another experiment, Atlas. Atlas is larger but less dense. CMS is more compact and dense.
How does it all work?
The LHC is like a huge racetrack. The protons go along in one direction, and then another beam of protons goes along in the other direction. Magnets bend them in a circular path. And most of the time these two beams are kept separated, except at four positions within this ring, where we have detectors with a different kind of magnet, which focuses the two beams together. And exactly at that point where this happens—where the two beams come together—is our CMS detector. The collision is happening right in the middle of the detector.
How many protons collide?
The beam has about 2,800 “bunches,” and a bunch has about 1,011 protons. So it’s a very intense bunch. But the collision point is very, very tiny, because the closer you can squeeze them, the more likely it is that an interesting collision will occur.
How often do you collide them?
The collisions are supposed to happen every 25 nanoseconds, which means—
Continuously? All day?
Yes, so 40 million times a second.
That’s so much data!
Yes, exactly. That’s the problem.
I thought you did it, like, once a month or something.
No. This is happening 40 million times a second and running over extended periods of time. Of course, I should clarify that for the past run, we ran at half that frequency. It was only 20 million times a second. But when we start taking data next year, it will be 40 million times a second. And this is the challenge. We just don’t have the kind of technology or the money to be writing out all of this data.

What does it mean to “write out” data?
Selecting and writing them to disk, so we can analyze it later. We need to cut down to a rate which is more manageable; I would say a few hundred events per second—at most, 1,000 events per second. So you want to go from 40 million times to 1,000. This has to be done in real time, and that’s where the trigger comes in. It’s literally a filter, or you’re “triggering” on interesting events. That’s where the name comes from.
Now I get it—so you set up the filter beforehand?
You set up the filter beforehand, and the filter fires every time an interesting event comes along. So that’s the trigger firing on something interesting.
So it sees something interesting, it fires, and then that data is written.
Right.
And you’re the one who figures out the triggers!
Yes! I lead the team that figures this out. It’s challenging, because whatever you say “no” to is literally going into the trash.
That would be a terrible feeling.
Yes. You want to make sure that all of the new physics, and the fun stuff, is not going into the trash. So we have a complicated trigger system. At the very first level, it makes some coarse decisions: Does this event have an electron? Does this event have a muon? Does the muon have a certain momentum? Does the electron have a certain momentum?
And the reason it’s looking for those things is because those things would be boring? Or interesting?
Muons and electrons are subatomic particles that are predicted to appear when certain other particles decay. We initially start with protons colliding with protons, so if energetic muons and electrons are produced, it indicates an interesting event. So the Level 1 part of the trigger has about three microseconds to make a decision. It doesn’t do detailed calculations. It doesn’t have that luxury. It just says, “This event seems interesting enough. I’m going to keep it.”
Then comes the second level of the triggering system, which is called the High Level trigger. And this is essentially algorithms, which are trying to make more of an informed decision. They have a little bit more time, about 200 milliseconds per event, and can do some more detailed calculations. They can obtain the momentum with better resolution. They can try to figure out if two muons that they see come from a certain particle, which has a certain mass. Of course, all of this assumes that we understand what the new physics will be, which we don’t. This is the part that keeps people awake at night.
You keep mentioning the “new” physics. What’s wrong with the old physics?
It goes back to what’s called the Standard Model of particle physics. It’s essentially a set of very elegant equations that describe our current understanding of the universe. So we know, for example, that there are these light particles: the leptons, electrons, muons, taus; and associated with each one of these is a neutral particle called the neutrino. We also know that there are generations of quarks: there is up, down, strange, charm, bottom and top—or beauty and truth, as they’re sometimes called.
We know all these very well. We also know that there are these forces, such as the magnetic force, the weak force, the strong force, and then the gravitational force. Now, each of these forces has an associated particle. One that is predicted, for example, is the graviton, which is a carrier of the gravitational force.
Now, going back to your question: Why are we interested in other theories? The problem is that the Standard Model leaves us with some things that are not explained very well. Firstly, gravity. The Standard Model doesn’t quite include gravity in this full picture. And the carrier of gravity, which is this hypothetical particle called the graviton, we haven’t seen it. So the Standard Model does not include gravity, and that is something which people are not happy about, naturally.
I can imagine.
Then the other two big mysteries. At the very creation of the universe, right after the Big Bang, we had equal amounts of matter and antimatter. However, everything we see around us is primarily matter. So where has all the antimatter gone? Then the other big issue is that a large fraction of the universe is what’s called dark matter. Now, what is dark matter, really? Again, the Standard Model doesn’t quite explain that.
So, to answer all of these big questions, you have to study these little tiny things.
Yes, these big questions are driving the kind of physics we’re doing. These new theories, which try to answer these big questions, have various predictions about new particles, so we are actively searching for these new particles.
Why do you like designing triggers?
It’s a lot of responsibility, making sure that you’re not making a wrong decision. So I’ve always liked challenges. And these are very, very complicated detectors. And things can naturally go wrong in some part of the detector, and the first place this shows up is at the trigger. If you have bad electronics in a certain part of the detector, it will, for example, just start firing all the time. And so you will see it because the trigger rates will just go spiking up.
And you’ll go, “Oh my God, we just found the new physics! Oh, never mind, we didn’t.”
Exactly. This has happened a number of times. You get very excited because you start seeing spikes, and then you find out that no, this was faulty electronics.
And then, for some reason, the people who work on the trigger are a certain kind of people. They are very passionate, they’re very dramatic, and the trigger meetings are dramatic places. People say, “I want certain kinds of triggers for my physics group, and you’ve got to give it to me or else I can’t do anything.”
I had no idea that it was so operatic.
[laugh] It’s always been like that. So it’s fun.
Comments & Discussion
Boston University moderates comments to facilitate an informed, substantive, civil conversation. Abusive, profane, self-promotional, misleading, incoherent or off-topic comments will be rejected. Moderators are staffed during regular business hours (EST) and can only accept comments written in English. Statistics or facts must include a citation or a link to the citation.