Scrambled Signals
Going deep into the brain to analyze the wiring at the root of autism
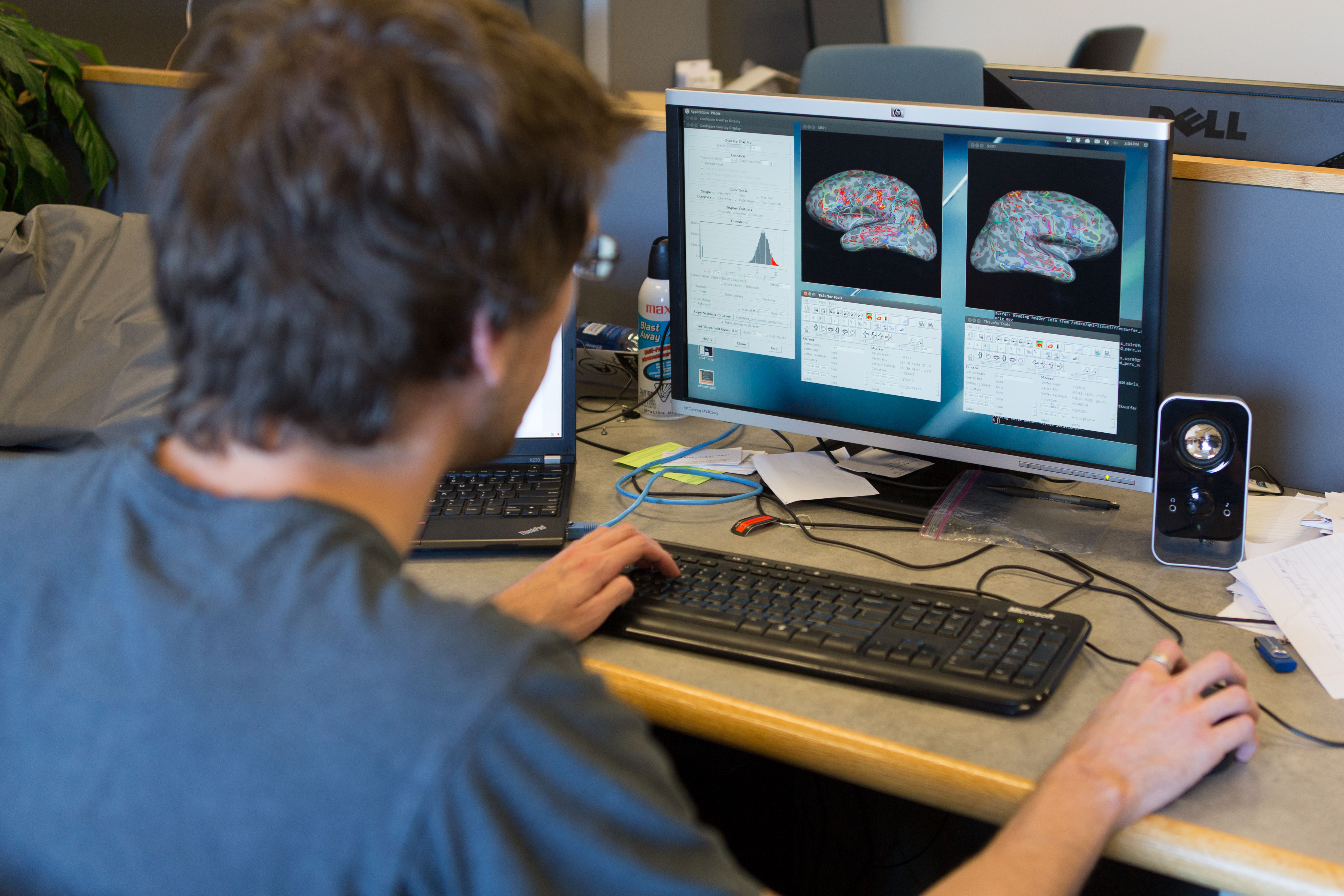
When you multitask, the front of your brain is performing all sorts of acrobatics. As you flit between reading this article and scanning your email, from listening to a colleague to swigging your coffee, your prefrontal cortex is controlling the many signals darting through your head—some don’t travel far; some do, following connections that stretch all the way back to your neck. Your attention glides smoothly from article to email, from colleague to cup.
This all assumes you don’t have autism. In 2010, Basilis Zikopoulos, a research assistant professor at Boston University’s Sargent College of Health & Rehabilitation Sciences, helped lead a study that found the prefrontal cortex of adults with autism is overloaded with connections, but that relatively few axons—the formal name for the brain’s wires—stretch from it to more distant regions. People with the disorder battle an overwhelming cacophony of signals at the front of the brain and relative silence throughout the rest.
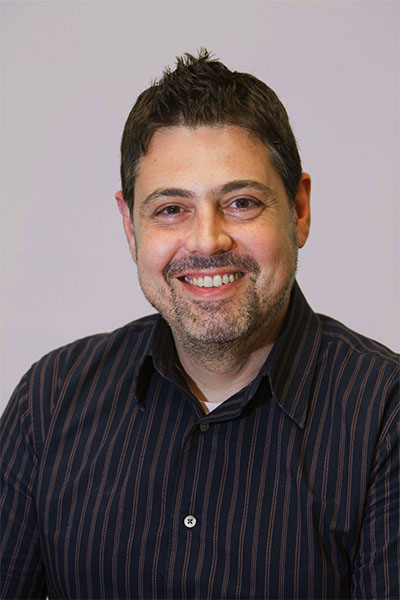
Zikopoulos points to a section of the prefrontal cortex that is particularly affected by autism. “The anterior cingulate cortex is implicated in many processes, but primarily in attention and emotions,” he says. When noise in this area becomes excessive, “it’s very difficult to direct attention. And when the long-distance connections are weak, you get poor communication between distant areas, such as the parts of the brain dealing with your senses.” The signals become scrambled, causing problems with attention and social and emotional interactions.
Having identified the distinctive connectivity of the brains of adults with autism, Zikopoulos wants to discover when—and how—the problems begin.
As part of a new National Institute of Mental Health–funded study, Zikopoulos will have access to 30 pediatric brains—15 from children who had autism, 15 from those who didn’t. He hopes to be able to build on the earlier findings to draw a timeline of autism, tracing its development and progression from before birth to adulthood.
For the next five years, Zikopoulos and a team of researchers at Sargent’s Neural Systems Lab, including the lab’s director, Professor Helen Barbas, will scan just about every square micrometer of the frontal lobes from those 30 brains. Using electron microscopes that can magnify a sample up to 100,000 times, researchers will be able to scrutinize not just individual axons, but details like the insulating layer surrounding them and the mitochondria—cell energy producers—inside them.
“I can see how neurons communicate with each other. We stain for specific proteins to see whether an axon or a neuron is inhibitory or excitatory; in other words, if it’s a stop or go signal in the brain,” says Zikopoulos. “A handful of labs in the world can look at the brain as a whole—all the way down to molecules and synapses. We’re one of them.”
There are many theories about what causes autism and when the disorder starts to distort the brain’s connections, but nobody knows for sure. Genes have been implicated, but are only responsible for about 10 percent of cases. It’s possible the brain might begin to misconnect very early in development—during neurogenesis, the very birth of our neurons—or perinatally when axons begin to grow.
“A handful of labs in the world can look at the brain as a whole—all the way down to molecules and synapses. We’re one of them.” —Basilis Zikopoulos
One of the reasons for this uncertainty is that it’s difficult to study the human brain in action. Scanning techniques such as MRI are imprecise—results have been compared to a map that only shows the brain’s superhighways, not its side roads. “It’s hard to connect behavior to molecular, neurochemical, or other features of the brain,” says Zikopoulos. “I would have to observe a person’s behavior, then take the brain out and slice it.”
The other reason for so much conjecture about how the human brain functions is that there just aren’t that many donated postmortem brains out there. The 30 brains Zikopoulos will have access to is, therefore, “a pretty high sample size for postmortem tissue studies,” he says. “We’ll be looking at different brain areas at different ages and stages of development.”
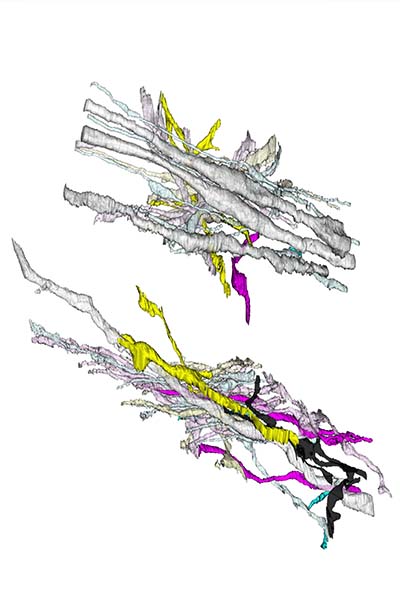
After five years, Zikopoulos expects to have a lot of data. Daniel Bullock, a BU professor of psychology and an expert in computational modeling, will help him visualize it. Bullock aims to build “behaviorally predictive computational models” that can simulate brain function and the impact of different developmental paths and drug interventions. Another BU psychology professor, Helen Tager-Flusberg, director of the University’s federally funded Autism Center of Excellence, will help connect the data back to the individuals’ clinical pathologies and diagnoses.
Zikopoulos calls his part of the research, “figuring out the fundamentals.” If he can better understand the neurochemistry and molecular features of different brain areas—as well as how they’re all connected—he can begin to pinpoint where and when the brains of people with autism veer off from the rest of the population. “If, for example, I find there is a specific protein that is different in the two populations, we might be able to design therapies, drugs, that will target that protein.”
In the 2010 study, Zikopoulos and the Sargent team concentrated on one potential rogue protein, growth-associated protein 43 or GAP-43. The protein, which he says is “expressed in a specific period of development,” stimulates the growth of axons in newly forming brains. In the anterior cingulate cortex of people with autism, it seemed to do too much stimulating.
“We need to figure out what makes someone more prone to autism and what environmental factors come in and make that happen,” says Zikopoulos.
To read more about Zikopoulos’s research, click here.