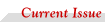 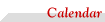 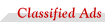 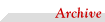
Mailing
List
Contact
Us
Staff
|
 |
Piecing
together the genomic puzzle
By
Tim Stoddard
 |

|
|
Charles DeLisi, Arthur G. B. Metcalf Professor of Science and
Engineering and senior associate provost for bioscience, has been
instrumental in putting BU at the forefront of genomic research.
Photo by Vernon Doucette
|
|
 |
Now that the human genome, that vast trove of genetic information encoded
in our DNA, is only a mouse-click away, researchers face the daunting
task of figuring out how tens of thousands of genes choreograph the life
and death of cells. Under the leadership of Charles DeLisi, Arthur G.
B. Metcalf Professor of Science and Engineering and senior associate
provost for bioscience, faculty in the College of Arts and Sciences,
the School of Medicine, and the College of Engineering are developing
new ways of understanding the complex machinery inside living cells.
“
We now have the full text for many genomes, but the text for the human
genome has not yet been completely parsed into words,” says DeLisi,
who is considered the father of the Human Genome Project. “When
it is — and we can reasonably expect to be 90 percent complete
in the next three to five years — we’ll have a parts list.
We’re
also developing the tools to understand how those parts interrelate.
It’s not like putting together a jigsaw puzzle or a 747. It’s
harder than that, because connections between the parts are
not fixed
— they change in response to the environment. The study and design
of such complex adaptive systems is a major research area of modern engineering.”
Following
is a look at some of the newest programs in genomic research at the University
and specific work being done by Boston University researchers.
Program
in bioinformatics
As genomics has changed the biological landscape,
Boston University has launched a graduate program to train rising scientists
for leadership
in the burgeoning field of bioinformatics. Founded in 1999 with a grant
from the National Science Foundation’s Integrative Graduate Education
and Research Traineeship Program, this University-wide program provides
a unique interdisciplinary perspective on the science, engineering,
medicine, and ethics of 21st-century cell biology. “To most of
us, bioinformatics is a way to understand the cell as a system,” says
DeLisi, the program’s director. “It’s the intersection
between computer science and genetics. It’s a way to identify
the functions of thousands of genes much more rapidly than we could
have in the past.”
The program comprises 50 faculty members, and
its thrust is integrating biology with information sciences and engineering.
But there is also
a core course exploring the legal and ethical issues emerging in the
field of biotechnology. Advanced mathematics and computation figure prominently
in the curriculum, which covers such current topics as genomic and proteomic
biotechnology, microarray engineering, and structural biology. “One
of the most beautiful aspects of this program,” says Simon Kasif,
an ENG professor of biomedical engineering, “is that it’s
producing a new breed of students capable of carrying out complex biological
experiments and analyzing them with sophisticated computational ideas.
In the past there were biologists who did pipetting, and statisticians
who did the analysis.”
The sequencing of the human genome was the “first
event when all biologists understood that they could not survive without
computers,” says
Sandor Vajda, an ENG professor of biomedical engineering and one of the
core bioinformatics faculty. “It is simply not possible to access
all of this genomic information in any other way. You need excellent
databases and very good interfaces for searching. As the computing speed
goes up, we will be able to answer much more complex questions than we
have in the past, going beyond simply searching the database.” The
computing resources at Boston University — such as the Biowulf
Linux Cluster, an IBM 128-node multiprocessor — facilitate the
massive number of calculations that arise when manipulating gene sequences
and protein
structures. For more information about the Bioinformatics Graduate
Program, see bioinfo.bu.edu.
Center
for Advanced Genomic Technology (CAGT)
The nascent Center for
Advanced Genomic Technology (whose acronym, CAGT, is a play on the
four bases of DNA, cytosine, adenine, guanine,
and
thymine) comprises the research core of the bioinformatics program.
Directed by DeLisi, it was founded in 2002 as a direct offshoot of
the molecular engineering research laboratory (MERL), which DeLisi
established in 1990 when he was dean of ENG. CAGT is divided into
four laboratories exploring different aspects of genomics and proteomics,
the study of the structure and function of the proteins that genes
produce.
Biomolecular systems laboratory
The biomolecular systems
laboratory (BSL), directed by DeLisi, is developing computational and
experimental methods for monitoring changes in proteins
and genes inside a cell as it responds to its environment. To this
end, DeLisi’s group has been improving DNA microarrays, a technology
now common in thousands of laboratories around the world. Often referred
to as a DNA chip, a microarray is usually a piece of glass about the
size of a microscope slide that is coated with a grid of thousands
of spots of DNA. Each spot contains millions of copies of short DNA
sequences, and a computer keeps track of what’s where. The beauty
of the array is that it makes it possible to measure the activity of
thousands of different genes at the same time.
“
The problem,” DeLisi says, “is that the arrays currently
don’t work very well, and more and more people are losing confidence
in them. Probably 90 percent of what we see on an array is in error.” He
and his colleagues have developed software to dramatically improve the
accuracy of the microarrays.
BSL researchers are also developing high-throughput
methods for identifying sets of proteins that are activated and deactivated
as conditions change
inside a cell. With assistance from BU’s Fraunhofer Center for
Manufacturing Innovation, BSL is forming Boston Array Technology, a company
that will produce a new kind of microarray for identifying thousands
of proteins using only the genome sequence of the organism being studied.
Flipping
the switch
Genes are blueprints for proteins, but proteins called
transcription factors are responsible for turning genes on or off by
attaching to
stretches of DNA called cis-elements, which are toggle switches that
tell the cell to express nearby genes. Zhiping Weng, an ENG assistant
professor of biomedical engineering, is developing computational methods
for mapping the precise locations of these switches in the genome,
and is also trying to find out what combinations of transcription factors
turn genes on and off. As the director of CAGT’s laboratory for
protein engineering (called Zlab, for Zhiping’s lab), Weng wants
to understand how proteins control the behavior of cells. “In
the human genome, there are maybe 30,000 genes,” she says, “and
for each one, we want to know what cis-elements are functional. The
ultimate goal is to find out for each gene how many transcription factors
are controlling that gene, when they become functional, and how the
cell is controlled in terms of transcription.”
Protein landscape
Transmitting a message from the surface
of a cell to its nucleus, where genes reside, involves a daisy chain
of interactions between messenger
proteins. Sandor Vajda is using high-speed computation to understand
how proteins physically interact with one another and with certain
small molecules (aka drugs). As director of CAGT’s structural
bioinformatics laboratory (SBL), Vajda is developing computational
methods for predicting which proteins will stick together, and what
the married proteins will look like as a couple. “Determining
the structure of two interacting proteins is extremely complex,” he
says, “because you have to generate billions of relative conformations
of the two proteins. And they’re not rigid bodies, so we have
to take into account that they are moving. To follow all of this movement
is an immense amount of computation.” Vajda also wants to find
small molecules that can cut in and interrupt the dancing pairs of
certain proteins. Studying the docking of small molecules is key to
the development of rational drug and vaccine design strategies.

|
 |
Using computational models of the human genome,
Zhiping Weng, an ENG assistant professor of biomedical engineering,
is searching
for the precise locations of so-called cis-elements — stretches
of DNA where proteins attach and turn nearby genes on and off.
Ultimately, she wants to understand how signaling proteins choreograph
the behavior of cells. Photo by Kalman Zabarsky
|
|
 |
|
Computational genomics laboratory
The genomes of over
100 species of bacteria are now easily accessed online, but in most cases
researchers do not know what over a third of the
genes do in the genome. “One of the great surprises of the genomic
revolution was discovering how little we actually know about organisms
that we previously thought we understood very well,” says Simon
Kasif. “We can identify the genes, we know they’re translated
into proteins, but we have no idea what they do.”
The main thrust
of the computational genomics laboratory (CGL), directed by Kasif,
who is also the codirector of CAGT, involves developing new
technologies for classifying undescribed genes into broad functional
categories. To do this, Kasif’s group compares genomes from different
organisms or different strains of the same organism. In this way, they
isolate interesting genes that are either changing quickly or not at
all, and they are able to infer the function of mystery genes. “There’s
been a tremendous push to understand what these genes might be doing,” Kasif
says. “All of the new genes recently discovered open up a whole
set of opportunities for discovering new diagnostic procedures or identifying
drug targets and vaccines.” The lab has focused on groups of genes
that appear to play a crucial role in infecting hosts, because they are
natural drug targets. In addition to identifying new genes and assigning
them biological functions, Kasif and his colleagues are building computational
frameworks that use experimental data to predict the behavior of cells
in normal or perturbed conditions, which promises to have an impact on
drug design and screening. For more information about CAGT and its
associated laboratories, see www.bu.edu/cagt.
|
 |