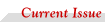 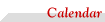 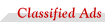 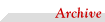
Mailing
List
Contact
Us
Staff
|
 |
Cancer
research at the cellular level
By
Brian Fitzgerald
 |

|
|
CAS Biology Professor and Department Chairman Geoffrey
Cooper worked with recent graduate Rahmat Muhammad (CAS’03)
on the regulation of a gene that plays a role in controlling
the life
cycle of cancer cells. Photo by Kalman Zabarsky
|
|
 |
Researchers on both the Charles River Campus and the Medical Campus
are probing the causes of cancer, studying its mechanisms, and increasing
our understanding of the pathways and the genetic changes in cancer cells — research that is leading to new approaches in prevention and treatment.
When cells refuse to die
CAS Biology Professor and Department Chairman
Geoffrey Cooper’s
research draws from cell regulation and development. In the early 1980s,
as an associate professor at Harvard Medical School, Cooper oversaw a
laboratory that was one of the first to discover human oncogenes, genes
that cause the growth of cancerous tumors. Now his work has led him to
study not how cancerous cells come to live, but how normal cells are
programmed to die — a program that has gone awry in cancer cells.
“
The failure of cancer cells to die is as important as the fact that they
divide too much,” says Cooper. New knowledge of the genes that
cause this phenomenon may help biologists create more effective treatments.
The problem with chemotherapy is that most of today’s treatments
are too general, killing every type of dividing cell. “If we can
target the genes that cause certain cancers,” he says, “we
can design new drugs that specifically attack cancer cells.”
Six
years ago, Cooper recruited Ulla Hansen from Harvard University. Now
a CAS biology professor, she has made fundamental discoveries in
the field of gene expression. Hansen studies the basic factors that control
the life and death cycles of cells, focusing on the role played by a
human protein known as LSF (late simian virus 40 factor). LSF is a transcription
factor — a protein that controls the expression of particular genes,
in this case a gene that produces an enzyme, thymidylate synthase, that
is key in the processes of DNA replication and cell division. Because
of the central role of thymidylate synthase in cell growth, it has been
used as a target for cancer therapy for many years.
Hansen is developing
novel methodologies to inhibit the action of LSF and hinder the production
of thymidylate synthase, causing the death
of cancer cells by apoptosis — the natural death process of cells.
Because LSF responds to changes in the cellular environment, such as
those caused
by hormones, she and her colleagues believe that it may play a central
role in helping the cell decide when to grow, stop growing, and die.
Another CAS biology professor, Thomas Gilmore, investigates how a protein
called REL may trigger cancers of the blood and immune system, such as
leukemia and lymphoma.
“
Mutant versions of specific types of proteins are implicated in most
human cancers,” says Gilmore. To mimic how the REL protein may
be involved in immune cell cancers, his lab has developed a model system
in which researchers use the human REL protein to cause a malignant change
in chicken blood cells cultured in petri dishes.
These in vitro experiments
not only establish for the first time a causal role for the REL protein
in human cancer, but they also provide a system
that can be used to understand how the REL protein causes malignancy
and to identify chemicals that inhibit its cancer-causing activity. Such
REL inhibitors are being developed in collaborative studies with CAS
Assistant Professor of Chemistry John Porco and are promising candidates
for more effective cancer treatments.
In a different approach, CAS Biology
Professor and School of Medicine Professor David Waxman is trying to
improve the effectiveness of chemotherapy
by delivering gene therapeutic agents directly to cancer cells. Waxman
and colleagues at Massachusetts General Hospital and the Dana-Farber
Cancer Institute licensed a new gene therapy approach that generates
an active chemotherapeutic drug directly at the site of the tumor target — effectively
turning the tumor into a drug factory.
The therapy is
based on P450, an enzyme that is necessary to activate the commonly used
cancer chemotherapeutic drug cyclophosphamide. Normally,
P450 is found only in the liver, and is responsible for transforming
inactive anticancer drugs into active forms, which are then transported
through the blood stream to cancer cells elsewhere in the body. Unfortunately,
this widespread distribution of an active drug causes many of the adverse,
toxic effects associated with chemotherapy. “By activating the
drug at the tumor site, P450-based gene therapy can potentially eradicate
tumors using lower drug concentrations, while drastically reducing damage
to healthy cells in the surrounding tissue,” says Waxman. The licensee,
UK-based Oxford BioMedica, is now in a second phase of testing the therapy’s
efficacy in treating breast cancer and other cancers.

|
 |
ENG Biomedical Engineering Professor Irving Bigio has developed
a novel diagnostic technique called optical biopsy, which is expected
to detect precancerous or cancerous tissue without invasive surgery.
Photo by Kalman Zabarsky
|
|
 |
|
Shining light on cancer
A variety of approaches being
investigated by researchers in the College of Engineering’s biomedical
engineering department is also showing promise in the fight against cancer.
Professor
Irving Bigio, who joined the department in 2001 after more than 25 years
as a scientific staff member at Los Alamos National Laboratory,
is a leader in the field of biomedical optics — the use of light
and optical technologies for diagnostic or therapeutic purposes.
Bigio
is developing a noninvasive diagnostic technique called optical
biopsy, which will allow physicians to detect malignancies in the colon,
bladder, cervix, or other organs immediately and without removing any
tissue. It will also provide surgeons with a tool to determine in the
operating room if they’ve left any cancerous tissue behind. The
technique will be used to identify cellular structural changes in tissue
based on its light absorption and scattering properties.
His interest
in noninvasive and minimally invasive diagnostics began 14 years ago
with a call from a gastroenterologist looking for a better
way to detect signs of early cancer. Using an endoscope, doctors would
look inside the colon of a patient suffering from bowel disease and conduct
random biopsies — sending tissue to a lab for examination under
a microscope. They could end up doing 30 or 40 biopsies on a patient
and still miss
the spots of early malignancy. The gastroenterologist wanted a “magic
laser” he could use with an endoscope, which would pinpoint the
location of precancerous or cancerous sites and somehow transmit the
information to a computer screen.
This request led Bigio and his colleagues
to develop the optical biopsy method, a technique using a fiber-optic
probe that can be passed through
an endoscope or catheter. The probe’s optical fiber shines white
light on, say, the lining of the colon or bladder, and an adjacent optical
fiber collects the light that the tissue emits. Since the cellular components
of cancerous tissue are different from those of normal tissue, they scatter
light differently. A computer compares the spectrum emitted from the
tissue being examined with spectral signatures in a stored diagnostic
algorithm (a mathematical recipe) to determine if any pathology exists.
Bigio
and his clinical collaborators have recently been awarded a $5.5 million
grant by the National Institutes of Health’s National Cancer
Institute for a five-year clinical translational study of the optical
biopsy system and related technologies that they have developed, and
it is hoped that this study can lead to real clinical applications in
a few years.
 |

|
|
MED
Biochemistry Professor Gail Sonenshein and her laboratory group
are studying breast cancer: (front row, from left) Elizabeth
Demicco (MED’04), Sonenshein, and Nora Mineva (MED’04);
(top row) Shangqin Guo (MED’03), postdoctoral fellow Karine
Belguise, research associate Sean Eddy, postdoctoral fellow Sebastien
Jeay, Vidya Chandramohan (MED’04), Claudia Hofmann (MED’04),
and Xiaobo Wang (MED’04). Photo courtesy of Gail Sonenshein
|
|
 |
Cancer’s environmental roots
Researchers in the School of Medicine’s
biochemistry department are studying how carcinogens and other environmental
factors influence
the way genes function, causing them to signal cells to reproduce uncontrollably.
Professor Gail Sonenshein, whose previous research has indicated that
drinking green tea may provide protection against breast cancer, is examining
the relationship between environmental factors and interrelated gene
systems that appear to be involved in the onset of breast cancer.
She
is examining how various mechanisms impact the activity of proteins in
the REL family, and how c-Myc proteins work to both inhibit and express
cellular activity. By better understanding the molecular pathways that
affect the expression of these genes, Sonenshein and her colleagues hope
to pave the way for the creation of biomarkers and powerful new cancer
therapies. The multidisciplinary study includes researchers from the
School of Medicine’s department of medicine and department of pathology
and laboratory medicine, and the School of Public Health’s department
of environmental health.
Aging and cancer
Barbara Gilchrest, a MED professor and chair of the
department of dermatology, has been investigating a mechanism that swings
into action when a specific
repeated sequence of DNA bases is detected in a cell — a mechanism
she and her colleagues hope will lead to new cancer prevention strategies.
Human cells can divide only about 20 times in a healthy adult. This
limit is associated with shortening of telomeres, the loop-like structures
at each end of the central, gene-containing portion of the chromosome.
Like all DNA, telomeres are double-stranded, but one strand ends in an
overhang composed of the six-base sequence TTAGGG, which repeats for
a length of approximately 150 to 200 bases. Thymine, adenine, and guanine
(T, A, and G) are three of the four building blocks, or bases, that make
up DNA (the fourth is cytosine, or C). The overhang is normally concealed
inside a loop structure at the chromosome end.
Gilchrest and her colleagues,
including Guang-Zhi Li, a postdoctoral fellow in dermatology and the
study’s first author, hypothesized
that as telomeres shorten in normal aging or are damaged by stress, ultraviolet
light, or carcinogens, the overhang sequences are exposed, setting in
motion a series of reactions causing the cells to stop replicating, or
even to commit suicide (apoptosis). To test this they introduced small
DNA fragments with the same TTAGGG sequence into cells in culture. They
observed that the fragments were rapidly taken into the cell nucleus
(where the chromosomes are located), and senescence (decline) or apoptosis
resulted.
“
If a cell is at high risk for becoming cancerous because of acute DNA
damage or simply aging, which exposes cells to environmental carcinogens
over many years, nature wants to protect the tissue,” says Gilchrest.
She and her colleagues propose that by triggering DNA damage responses
without actually damaging DNA — by providing telomere-mimicking DNA
fragments — they may be able to develop new treatments for cancer,
a condition in which cells seem to have lost their ability to senesce
or
otherwise recognize and respond to abnormal DNA.
Cancer Research Center
Basic scientists at MED’s Cancer Research
Center collaborate with clinicians to expand research horizons. For example,
Douglas Faller,
a professor of medicine and the center’s director, has been working
with MED Associate Professor Susan Perrine to develop a gene-based therapy
to kill tumor cells associated with Epstein-Barr virus (EBV), one of
the most common human viruses. The thymidine kinase (TK) gene can be
deactivated by the antiviral drug ganciclovir, but since EBV-associated
tumors normally don’t express viral TK, they don’t respond
to treatment with ganciclovir. To overcome this, the researchers are
investigating the use of the drug arginine butyrate, which can induce
the TK gene in these tumors to make them susceptible to ganciclovir.
Early
detection of lung cancer
In another study, Jerome Brody, a MED professor
and a widely recognized authority on the molecular and cell biology of
the lung, and Avrum
Spira, a pulmonary faculty member who trained in the Bioinformatics
Graduate Program, are working on a way to diagnose lung cancer that
is less invasive than thoracic surgery.
There are no effective screening
tests for early diagnosis of lung cancer, which claims more than 150,000
lives each year in the United
States,
exceeding the combined mortality from breast, prostate, and colorectal
cancers. A major hurdle in developing an early detection screen for the
disease is that present diagnosis requires invasive surgery to remove
tissue from inside the lung for biopsy. However, Brody, director of the
Pulmonary Research Center, and codirector of the Cancer Research Center’s
Lung and Airway Cancer Research Program, is developing a method that
requires only a minimally invasive brushing or scraping of the pulmonary
airways.
Brody is collaborating with Affymetrix, Inc., a company that
produces GeneChip microarrays. Using these microarrays, he is developing
a method
to compare gene expression signatures of harvested airway cells against
known pathogenic signatures. He expects that this new technology will
allow doctors to detect lung cancer in its early stages, help them better
monitor the course of treatment, and make better decisions about prognosis
and therapy. “The preliminary results of our initial microarray
study were very encouraging,” Brody says, “and we see great
potential for introducing microarray-based tools into the clinical routine.”

|
 |
Members of MED Biochemistry Associate Professor
Zhi-Xiong Jim Xiao’s laboratory group studying tumor suppressor protein
p53: (bottom row, from left) Donny Li-Fan Chang (MED’04),
Jim Xiao, and Hongwu Zheng (MED’03); (top row) research associate
Jinan Yu, research associate Patima Sdek, Duc Tran (MED’04),
Haoqiang Ying (MED’04), and research assistant Kui Tian.
Photo courtesy of Zhi-Xiong Jim Xiao
|
|
 |
|
Putting
on the brakes
In MED’s biochemistry department, Associate Professor
Zhi-Xiong Jim Xiao and his colleagues are studying the function and regulation
of tumor suppressor protein p53.
Named “Molecule of the Year” by
the journal Science in 1993, p53, and its associated gene,
literally puts on the brakes, stopping cell division
when it senses that a cell’s DNA is damaged and the cell is likely
to reproduce in the uncontrolled manner that leads to cancerous tumors. A
defect
in the p53 gene has been implicated in an estimated 60 percent of human cancers,
including those of the breast, lungs, liver, skin, prostate, bladder, cervix,
and colon. Although much has been learned about how p53 functions -- and
malfunctions — the mechanisms that lead to its activation remain largely
unclear.
Xiao is beginning to put that piece of the puzzle together. His work,
published in the October 24, 2002, issue of the journal Nature,
reveals that when DNA
is damaged, there is an interaction between p53 and Pin1, one of a group
of enzymes present in the body that initiates the rearrangement of protein
conformation.
He further found that the interaction occurs at very specific binding sites
on the protein, known as Ser33, Ser315, and Thr81. In cells lacking Pin1,
p53 does not react effectively when DNA is damaged, allowing the damaged
cell to
proliferate out of control.
Better understanding of this mechanism, Xiao proposes,
may lead to the development of therapeutic drugs that can intervene and enhance
the ability of p53 to
stop a malignant cell that is out of control.
|
 |