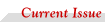 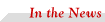 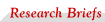 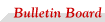 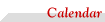 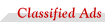 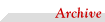
Mailing
List
Contact
Us
Staff
|
 |
Visionary
science
CAS prof peers into the brain’s visual cortex
 |

|
|
Eric Schwartz,
a CAS professor in the department of cognitive and neural systems,
is using this functional magnetic resonance
scanner at the Massachusetts General Hospital’s research
facility to peer into the visual cortex. The real-time images are
shedding light on how the brain makes sense of the visual world.
Photo by Bruce Caplan
|
|
 |
By
Tim Stoddard
It’s sort of like looking down a hall of mirrors: trying to see
how the brain sees while it’s, well, seeing. That’s what
Eric Schwartz, a CAS professor in the department of cognitive and neural
systems, is doing, harnessing the power of functional magnetic resonance
imaging (fMRI) to watch the visual cortex in action. The results may
soon not only shed light on how the brain makes sense of the visual world,
but also help improve the power and accuracy of fMRI technology.
When
light lands on the velvety surface of the retina, specialized cells go
to work like a legion of telegraph operators, encrypting the image
as electrical impulses that zip along to the primary visual cortex, called
V1 by researchers. Neuroscientists have known for 50 years that the brain
arranges these signals in a kind of representational map, with each point
on the retina routed to a unique point in V1. Researchers think this
map is then copied onto 30 to 40 different areas within the visual cortex,
each of which interprets a different aspect of the image, such as colors,
edges, and motion. But with his colleagues at CNS, Schwartz has done
recent computational work that suggests the structure of the brain is
simpler and more elegant, processing images in only a few compartments.
“
This model would change the whole concept of how the cortex is organized,” Schwartz
says. “It’s as if in astronomy you didn’t have a concept
of a galaxy, but only had one for a solar system. And then somebody came
in and said, ‘Well, actually there’s a higher level of structure
that you didn’t see before.’”
With a $2 million grant
from the National Institute for Biomedical Imaging and Bioengineering,
a new branch of the National Institutes of Health,
Schwartz and Bruce Fischl (GRS’97), an assistant professor of radiology
at Harvard Medical School, are using fMRI to investigate the primary
visual cortex in unprecedented detail.
Magnetic vision
In the 1960s neuroscientists began using
implanted electrodes to study nerve activity in the visual cortex of
macaque monkeys. In the standard
experiment, the monkeys were shown a bull’s-eye pattern of white
lines on a black background. The researchers hoped to find a mathematical
function describing how the brain takes in the optical information
and distributes it within the primary visual cortex, but they found
no clear patterns.
As a postdoc in 1976, Schwartz revisited their data
and found that a complex logarithm describes the visual mapping fairly
well. It turns
out to be the same function for calculating the geometry of a magnetic
field. When you drop iron filings over a bar magnet, they spread out
in an hourglass shape along the axis of the magnet. “The mathematical
function that plots out the retina-to-cortex mapping is the same as that
which tells you how to plot out the field lines of a magnet,” Schwartz
says. “In some sense, the physical world is following the simplest
possible idea, and so is your brain.” He emphasizes, however, that
there are no bar magnets in our brains. “It’s the same mathematics,” he
says, “but it isn’t the same phenomenon.”
With CNS
colleagues, Schwartz recently did a detailed analysis of several topographic
maps described by other researchers, and found that they
have almost the same mathematical structure as V1. Their models suggested
that instead of 40 distinct topographic maps, there were only 2 or 3
separate “super-maps,” with many submaps nested within them
like Russian dolls.
Hardware tweaks
While this is still theoretical work,
Schwartz says, it gives researchers a new tool for understanding fMRI.
First developed about a decade ago,
fMRI works in real time by measuring oxygen levels in the blood throughout
the brain, indicating which neurons are getting busy and consuming
oxygen. But fMRI and MRI are only about 95 percent accurate; there
are minor distortions in the images that are trivial in most clinical
applications but problematic for precise measurements.
Schwartz plans
to use the mathematical model he’s developed as
a benchmark, and compare it to what he actually finds on the fMRI scans,
using ever more powerful fMRIs at a Massachusetts General Hospital research
facility. “You can’t really fix the imaging if you don’t
have a benchmark for what correctness is,” he says.
What kind of
biomedical progress can we expect from an even more sophisticated MRI? “The
consequences of improving imaging quality are hard to predict,” Schwartz
says. “In the history of science, pushing
the measurements further and further has been where progress comes from,
and I think the instrumentation drives the science. When it gets better,
the science gets better.”
|
 |