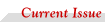 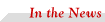 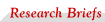 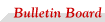 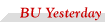 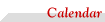 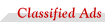 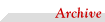
Contact
Us
Staff
|
 |
Whole
lotta shakin’
Major Alaskan quake
rocks alum, seismologists
By
Tim Stoddard
For most people, it would have been the wrong place at the wrong time,
but not for Josh Stachnik. On November 3 at 1:12 p.m., Stachnik’s
pickup truck began to dance a jig across a remote stretch of the Denali
highway. Stachnick (GRS’02), a staff seismologist with the Alaska
Earthquake Information Center, and two colleagues had been deploying seismometers
that morning along the dirt highway in the hope of recording aftershocks
from a magnitude 6.7 earthquake that had rattled the region on October
23. What they got was much more than an aftershock.
“I had just driven through a big field of potholes,” Stachnik
says, “when it felt like the truck was bucking me from the back
end. It started jumping all over the road. I thought I had a flat tire
or had lost a wheel or something so I stopped. I got out and realized
that the trees were flopping back and forth and the truck was still swinging
in the breeze.”
For the next three minutes, Stachnik felt the earth move under his feet
as the most powerful earthquake recorded on the planet so far this year
unleashed its seismic wrath across that sparsely populated region of Alaska.
Not only was Stachnik one of the closest human beings to the epicenter
of the magnitude 7.9 temblor, he certainly was the closest geologist.
According to Geoff Abers, a CAS associate professor of earth sciences
and Stachnik’s former thesis advisor, it was the largest earthquake
to occur on land in the United States since 1857.
Centered approximately 75 miles south of Fairbanks on the Denali fault,
the quake triggered countless landslides, but resulted in only minimal
structural damage and amazingly few injuries. The 800-mile-long Trans-Alaska
pipeline, which carries one million barrels of crude oil a day from Alaska’s
North Slope to the lower 48, was shut down for several days after the
quake broke a half-dozen energy-absorbing supports.
Most geologists would give their eyeteeth to have been in Stachnik’s
shoes that quiet Sunday afternoon, experiencing the power of an M7.9 in
a safe location. And the seismology community is now clamoring to see
the data from the instruments that were in the right place at the right
time. Until now, there have been only a few instances where seismometers
were positioned close to the epicenter of a major earthquake, says Rachel
Abercrombie, a CAS assistant professor of earth sciences, who studies
the mechanics of earthquakes.
“The Denali quake was a big deal,” says Abercrombie. “The
problem with our field is that we always want to have observations of
earthquakes within a few kilometers of their starting point, and we can
never predict where that will be. I think there are many seismologists
who are downloading data from this quake right now, and if there are some
nice recordings — which is perhaps what we’ll get from Josh’s
work — then they could be really useful.”
Stachnik says that it’s too soon to assess the quality of this data
because seismologists in Alaska are still scrambling to analyze their
recordings and to deploy additional instruments to catch aftershocks.
But the seismologists at the University of Alaska, Fairbanks, believe
that the data from Stachnik’s instruments will shed light on some
of the long-standing questions about earthquakes.
 |
 |
|
 |
|
The
motion of the ground along the Denali fault was evident along roads
such as this one. Photo by Akihito Ito |
|
 |
One of the main questions of interest to Abercrombie and other seismologists
is how big earthquakes get started. Since the mid-1990s, geologists have
debated two possible models. In the cascade theory, a major quake is triggered
by a series of smaller ones that set off a chain reaction along the fault.
The baby quakes can happen anywhere along the fault at any time, making
it virtually impossible to predict when and where the big one will occur.
An alternate theory called the nucleation model has been proposed by some
seismologists. In this scenario, pressure builds along a region of the
fault until the two sides begin creeping past each other at a weak point.
The slow creeping then accelerates into a rapid slip that releases the
earthquake’s energy.
Abercrombie has not yet perused the data from the November 3 quake, but
she says that after glancing at data collected from seismometers in California,
it looks like a small event immediately preceded the big quake. If this
prelude has the seismic signature of a normal small earthquake, it would
support the cascade model, she says. If it’s a peculiar creeping
signal, however, then it will support the nucleation model.
The seismic waves from the quake reportedly sloshed lakes in Louisiana,
Abercrombie says, and in Harvard, Mass., the waves shook a seismometer
back and forth by about four centimeters. “The seismometers will
be able to pick up the surface waves of this earth-quake on their first
trip around the world,” she says, “then their second and third
trips.” It takes about three hours for the surface waves to circumnavigate
the globe. “The earth is essentially ringing like a bell after an
earthquake of this magnitude, which enables people to use the seismograms
to do all sorts of interesting things, like find out what’s in the
inner core.”
In the meantime, seismologists will begin poring over the data collected
from the Denali quake. Abercrombie says that there are already plans to
devote a special session to this earthquake at the fall meeting of the
American Geophysical Union. And for once, seismologists can express their
enthusiasm over a big earthquake with a clear conscience. “The nice
thing about this quake is that we can legitimately get excited because
there were no serious casualties,” she explains. “So often
in an earthquake like this, it’s a mixture of enthusiasm and tragedy.
Now we can focus on the amazing event and not have to think about the
victims of collapsed buildings.”
|
 |